The location of this earthquake seems a little odd because North Carolina is about as far as it’s possible to get from an active plate boundary – thousands of km from the mid-Atlantic spreading ridge to the east and the San Andreas/Cascadia strike-slip subduction combo to the west.
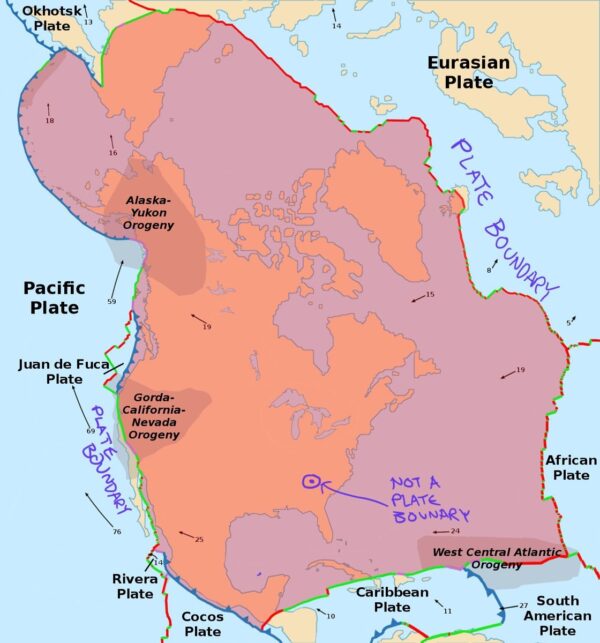
The whole point – and power – of plate tectonics is that most geological activity, including earthquakes, occur at the boundaries of the Earth’s tectonic plates. Those plates are constantly moving and rearranging, but they are rigid enough to do without changing shape.
However, it is more accurate to say that they do so without changing shape much. “Intraplate” earthquakes like this one, or the M5.8 that shook Virginia in 2011, show that in reality, the interiors of plates can buckle and stretch. It just happens at much, much slower rates than at their boundaries. No matter where we are, the crust under our feet is always under stress. In the eastern US, the main source of horizontal stress is “ridge push” from the mid-Atlantic ridge, which has a roughly NE-SW direction.
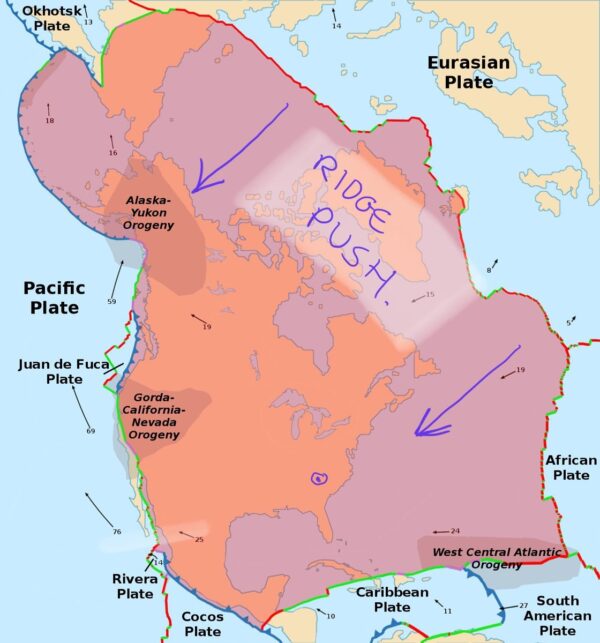
Normally, the interior of a plate is strong enough to take this stress, and transmit it onwards. But not always! The exceptions mostly occur in continental crust, which hangs around accumulating tectonic damage – faults & fractures – that make it weaker. Several times in the past billion years or so, this part of North America has been much closer to a plate boundary than it is now. Most obviously, the Appalachians are a testament to a continental collision about 350 million years ago.
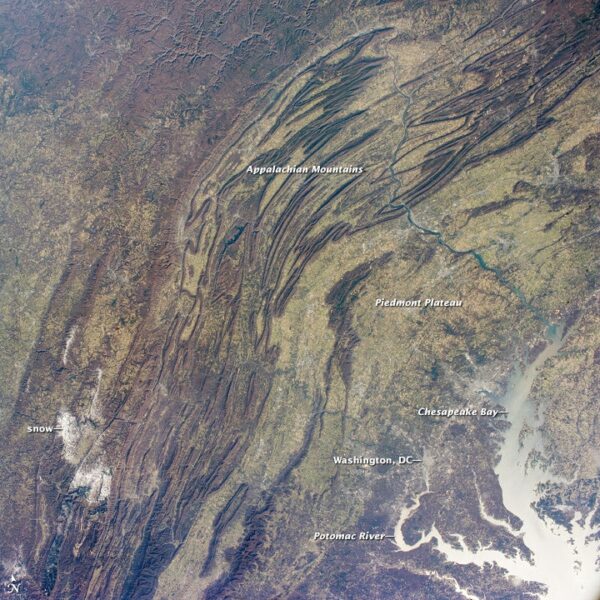
But after the Appalachians formed, this region was stretched by the rifting that eventually formed the Atlantic Ocean; and the older rocks that the Appalachians is built from record earlier collisions & rifts. These past brushes with plate boundaries have left plenty of faults that could be reactivated by the forces currently stressing the tectonic plates.
So we have stress, and structures that can potentially accommodate that stress. The focal mechanism indicates NE-SW compression on a reverse fault.
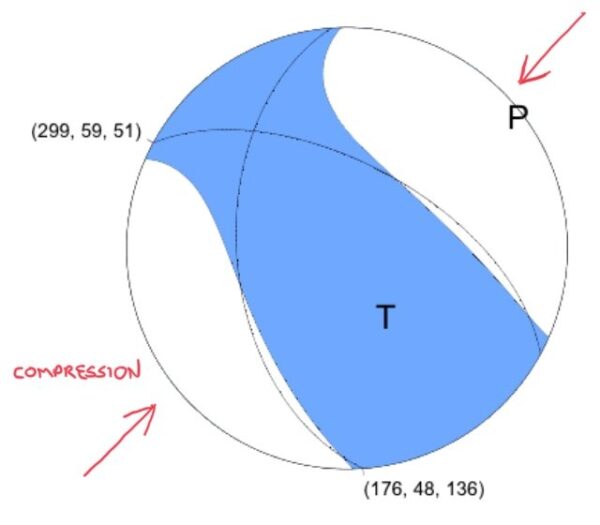
The direction of compression is consistent with the expected stress direction in this region due to ridge push. But as most of the old faults and structural boundaries in this region are also oriented NE-SW – the trend of the Appalachians – we might expect strike-slip faulting on a reactivated fault trending parallel to the stress direction, which seems to be a common type of earthquake in this region, rather than thrusting at right angles to the general regional trend.
So yesterday’s earthquake was a bit of an outlier. One possibility comes from the fact that this is not a perfect double couple mechanism. It could be due to a problem with data coverage, or it could indicate that something more complex, involving multiple faults with different orientations, is going on. Zooming out, however, it seems that compressional intraplate faulting is actually quite common to the east of the Appalachians- see the red region in the figure below.
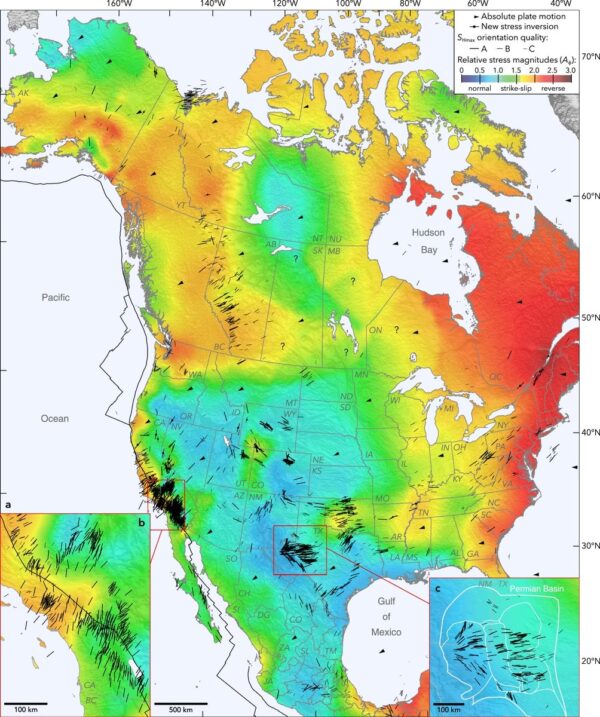
The basins created in this region by continental rifting as the Atlantic started to open in this region were linked together by NW-SE trending transform faults and lineaments (at least in Pennsylvania – I’m less familiar with subsurface structures in North Carolina). Perhaps one of these is being reactivated – at least to me, this seems more likely than entirely new faults forming.
Anyway, to sum up:
- we expect earthquakes like this every so often.
- this one is at least broadly reflecting the known regional stresses.
- it is a little bit weird, but intraplate earthquakes tend to be an idiosyncratic bunch.
[this post was collated from this twitter thread]
Nice plan for content warnings on Mastodon and the Fediverse. Now you need a Mastodon/Fediverse button on this blog.