Not much may have made it onto the blog, but it’s been a busy year for both Anne and Chris in 2017. Here’s a brief summary of what we’ve been up to – with pretty pictures where appropriate.
Spring:
Chris taught Structural Geology for the first time, and softened the mind-warping effects of structure contours and steronets by taking his students on a field trip to map some of the spectacular folds found in the Valley and Range of the Appalachians of West Virginia. He also taught the introductory How the Earth Works course.
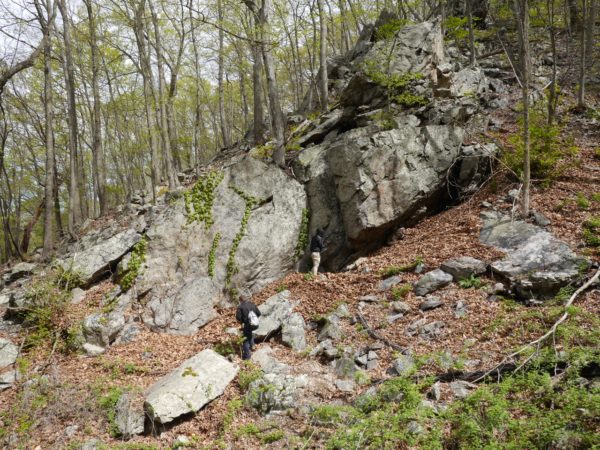
Some of Chris’s students getting a strike and dip off the distinctive Oriskany sandstone. Now where has the other fold limb got to? Photo: Chris Rowan, 2017.
Anne taught Watershed Hydrology and took her students on a field trip to the Shale Hills Critical Zone Observatory and the Johnstown Flood National Monument. She also survived her first admissions season as Graduate Coordinator for the department and her first time chairing a faculty search committee.
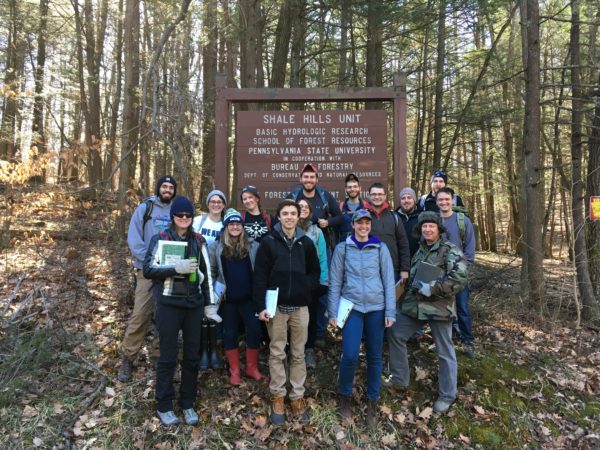
Kent State Watershed Hydrology students at the Shale Hills Critical Zone Observatory, home to one of the most intensely instrumented catchments in the eastern US. Photo: A. Jefferson, 2017.
Chris graduated his first MS student Matt Harding, whose thesis focused on the role of basement structures in controlling salt tectonics beneath the Allegheny Plateau.
Anne’s postdoc Pedro Avellaneda published a paper modeling the hydrologic response to a green infrastructure retrofit. This paper was built on our earlier experimental work on the project. With the model, we were able to isolate the effects of the bioretention cells versus other components of the retrofit, to identify the effects on the overall water balance of the catchment, and to quantify the effects of the green infrastructure on flows at specific probabilities (e.g., the “2-year” flow). Anne was really happy to have this be her first paper co-authored with her postdoc and her first paper where an undergraduate (REU) student was an author.
Chris’s undergraduate student Joe Wislocki presented some preliminary results of his analogue modelling of the development of the Pennsylvania Salient in the Appalachians at the GSA sectional meeting in Pittsburgh. Anne and her students gave talks in the urban hydrology session and a poster in the post-glacial rivers session at the same meeting.
Summer:
Anne, Chris, and family had another epic multi-national holiday. First, we spent a few (unusually hot) days hiking in England’s Peak District (a place we’ve enjoyed before). Then Anne headed for the HydroEco 2017 conference in Birmingham, where she gave a talk, sweltered in the heat, and saw the University of Birmingham’s Free Air Carbon Enrichment (FACE) experiment. Meanwhile, Chris and TerraTyke explored toddler-friendly spaces in Essex – mainly places on the (slightly cooler) coast.
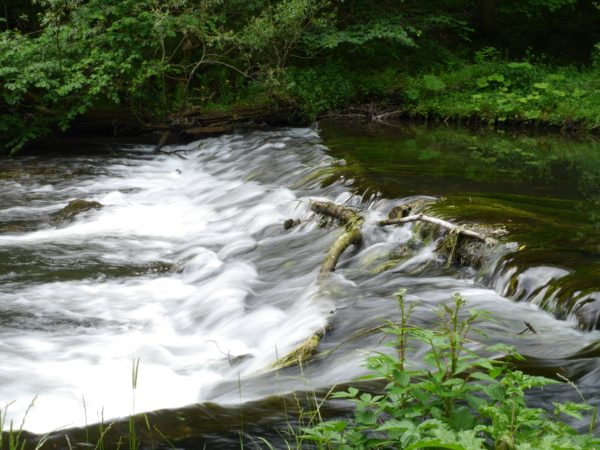
A pretty – but highly unnatural – weir along Dove Dale in the Peak District. Photo: Chris Rowan, 2017.
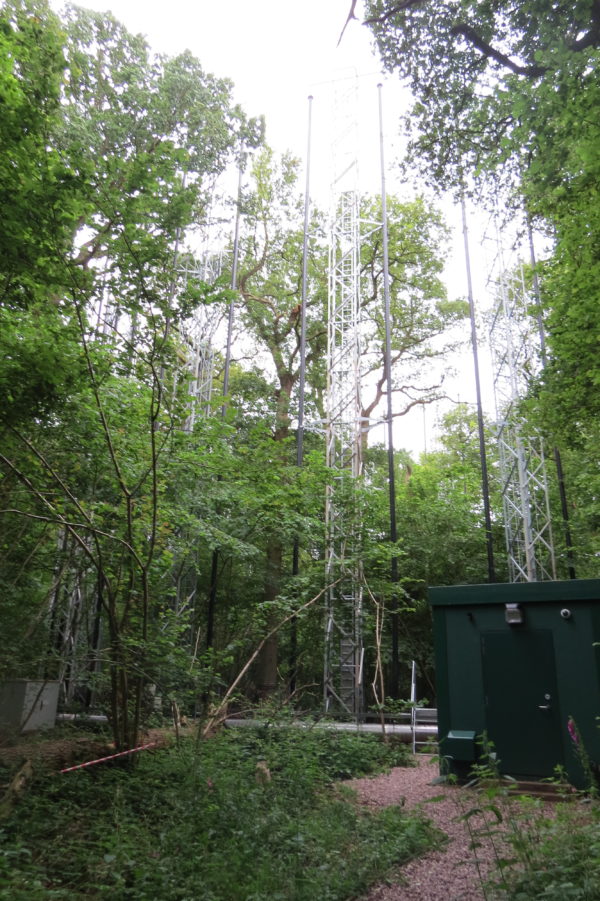
University of Birmingham FACE experiment in action. Carbon dioxide is blown out of the ring of towers and the effects on the vegetation, soil, and and hydrology are studied. Photo: Anne Jefferson, 2017.
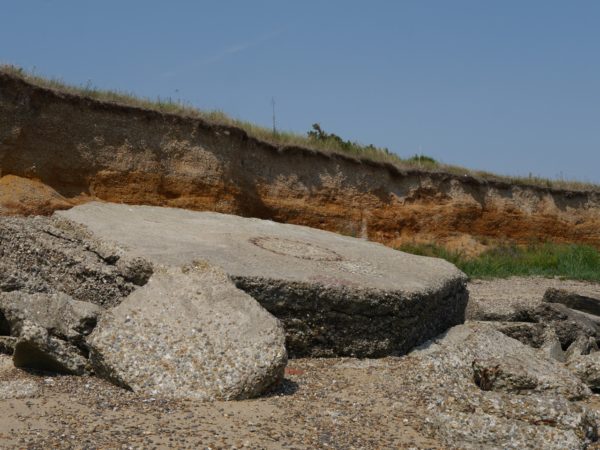
Erosion of WWII pillboxes and Pleistocene gravels on Mersea Island, Essex. Photo: Chris Rowan, 2017.
Our next stop was Iceland. What can we say? It’s geologist paradise and our streak of unusually hot and sunny weather continued. (Much more welcome in Iceland than the UK, Anne says.) On our first day, we did the famous Golden Circle stops of Pingvellir, Geysir, and Gulfoss, but at our own pace.
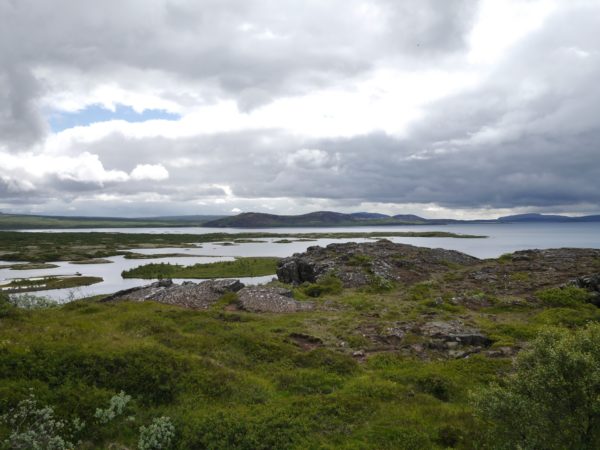
Standing on the North American Plate, waving at the Eurasian plate: looking over the edge of a rift fault at Pingvellir. With bonus pahoehoe lava flow tops in the foreground. Photo: Chris Rowan, 2017.
Then we pushed a little further east, where highlights included a ‘Super Jeep’ excursion up into the highlands to explore the vents of the 1783-4 Laki fissure eruptions, and a ferry ride to the Vestmannaeyjar archipelago, where we explored the effects of a much more recent eruption on the island of Heimaey
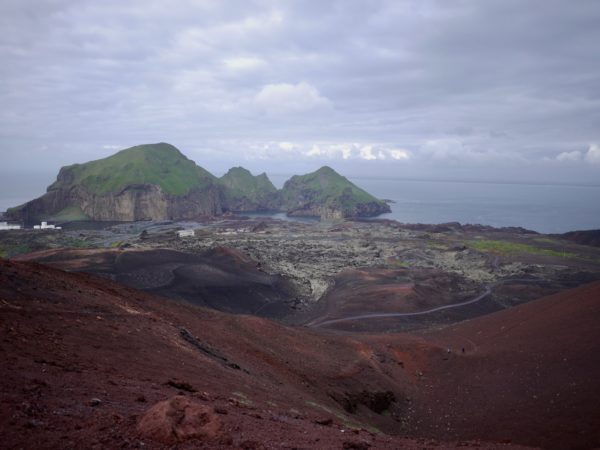
Looking down on the 1973 lava flow that almost blocked the harbour on Heimaey, from its source: Elfell volcano, also newly formed in the 1973 eruption. Photo: Chris Rowan, 2017.
Our summer tour concluded with a few relaxing days in southern Minnesota, enjoying the topography of the Driftless Area and spectacular views from Anne’s brother’s ridgetop farm.
In August, Chris spent a week at working with colleagues and students on Utah’s Markagunt landslide. This is the largest known terrestrial landslide, formed by the sector collapse of the Marysvale Volcanic Field about 20 million years ago. Chris is contributing to a student Master’s project that aims to constrain the timing and mechanism of landslide’s emplacement using paleomagnetic and rock magnetic techniques.
To round off our summer travels, we also took a weekend trip to the pretty, but unsurprisingly very crowded, Hocking Hills region in southern Ohio.
Fall:
Chris taught Geophysics and How the Earth Works (again), as well as a first year experience seminar. Geophysics is focussed on developing understanding of geophysical signals through forward modelling exercises in Excel and also in Python, using Jupyter interactive notebooks; the final project sees students working in teams, with a fixed “budget” to spend on data acquisition to identify the subsurface geophysical features of a mystery field area.
Anne was on a “research and creative activities leave”, so she was on a mission to get papers completed and new projects spun up.
Anne and 5 colleagues published a review and synthesis paper on what we know about the cumulative effects of stormwater management at the catchment scale. In this paper, they identified 100 studies from around the world, using field and modeling techniques, that had worked on elements of this problem. Synthesizing the studies, they identified concepts for which we have a reasonably good understanding of what happens as a result of stormwater management versus hydrologic processes and types of infrastructure for which we have big understanding gaps. They also identified the next needed steps in this research area. (Ironically, the paper was born out of a rejected proposal, where the reviewers doubted there were sufficient papers published to do such a synthesis. 100 studies later, Anne thinks they were wrong.)
With colleagues from the College of Public Health, led by Anne contributed to a paper on the prevalence and typing of Staphylococcus aureus at public beaches in northeastern Ohio. Tara Smith and her postdoc Dipendra Thali led the effort, which was born out an undergraduate (REU) project. Anne’s piece focused on the association between treated wastewater effluent in the lakes and the abundance of Staph. The two inland lakes that we sampled that had no wastewater also had less Staph in the water and sand samples. There’s a lot more to work out in terms of mechanisms, but this paper contributes a unique dataset to spur further research. The paper was published in AGU’s new open access GeoHealth journal and is free for anyone to read.
In addition to the papers, Anne also lead a proposal to NSF’s Environmental Sustainability program (fingers crossed) and contributed to one focused on hydrology education (more crossed fingers). She also enjoyed working with her team of graduate students on some new projects, with more to say in those areas in 2018 and beyond.
During fall semester, it felt like either Anne or Chris was always traveling, but never at the same time.
First, in September, Chris returned to southern Utah to participate in a GSA Thompson Field Forum on the Markagunt slide. He wins a point for earliest snow of the season.
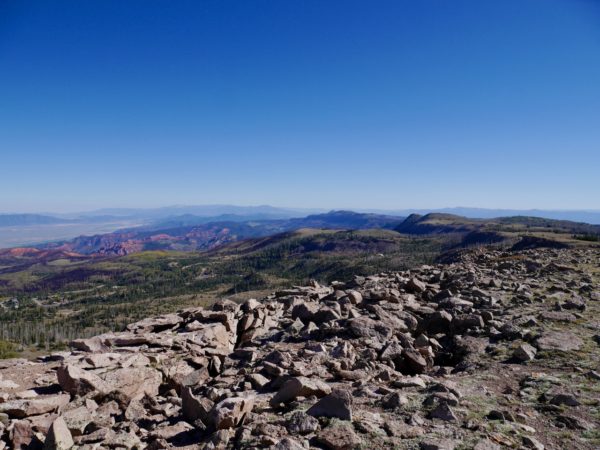
It’s really difficult to capture the scale of the Markagunt landslide, but in this view, we’re standing on the landslide (and not at the end) looking back towards its source, and everything you see in this picture is landslide. Photo: Chris Rowan, 2017
Then, in October, Anne took advantage of not teaching and attended both the GSA meeting in Seattle and a 4 day field trip over the North Cascades (in the snow) and through some Missoula megaflood carved landscapes. Then, she and her students gave talks and posters at the meeting itself. Anne’s first GSA meeting was in Seattle in 2003 and it was great to be back as a grown-up.
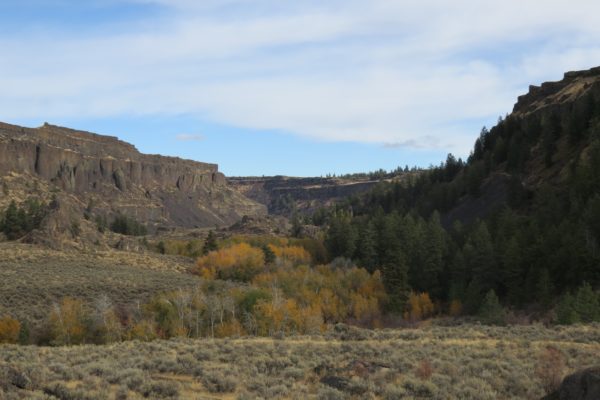
Like the mega-landslide Chris visited, it’s hard to pick a photo to capture the scale of Earth history’s largest floods. This is a lovely side canyon off of Grand Coulee, carved by one or more of the floods that predate the opening of Grand Coulee itself. Even 90 years after J. Harlan Bretz started to tell the story of the floods, there’ still so much to be figured out about exactly how and when each feature of the landscape formed. Photo by A. Jefferson.
In November, Anne spent a few days in Boulder, Colorado attending a workshop on promoting diversity and inclusion in the geosciences, through exercises focused on implicit bias, microaggressions, bystander intervention, and gatekeeping functions.
Chris then jetted off to Hong Kong for a friend’s wedding, and he also took the chance to (briefly) hike and ferry amongst the volcanic landscapes of the islands.
And in December, Anne and Chris both stayed home for grading, cookie baking, and enjoying the mercurial nature of Ohio winter weather. We look forward to more adventures in 2018.
Nice plan for content warnings on Mastodon and the Fediverse. Now you need a Mastodon/Fediverse button on this blog.