I admit it. I’m geocentric. Not in the old-fashioned sense, I’m not that eccentric. I don’t believe the earth is the physical centre of the universe, but it certainly feels that way. The universe, space, the wonders of the solar system are all very well, but emotionally they are all ‘out there’ somewhere. A Twentieth Century geological education contributed to this – everything on the earth was explained by something else on the earth. For example we were taught about the increasingly odd forms of late Cretaceous ammonites. The implication was that their extinction was due to them becoming decadent and depraved, like the late Roman Empire. When the theory that they and the dinosaurs where killed by a meteorite impact arrived, it felt somehow unnecessary (and yet now seems so obvious).
Which is by way of justifying why my response to Dana Hunter’s Accretionary Wedge call for ‘out of this world’ posts on exogeology has its feet planted firmly on terra firma. One of the most interesting strands of studies of this planet in the last 20 years has been discovering the many terrestrial features caused by external influence. Often ‘it came from outer space’ is science fact, not science fiction.
Meteorites are the most dramatic way in which ‘space’ affects the earth. Lumps of rock and/or iron hitting the earth at cosmic velocities (more than 11 kilometres a second) leave some dramatic traces.

Meteor Crater, Arizona. Courtesy of Scott Tanis on Flickr. http://www.flickr.com/photos/8376919@N02/3905452932
What is striking about meteor craters on the earth is how few there are. There are so many craters on Mars that studies of its geology use their areal density to estimate the age of the surface. A bit like people’s skin, old craggy areas look different to smooth new ones. By contrast, there are fewer than two hundred impacts recognised on earth. Partly this is due to a thicker atmosphere, mostly due to high rates of erosion and geological activity and partly because there are many more traces of impact left to be found.
Most large round features on earth have been investigated. Some small craters no doubt remain to be found but there are other ways to find traces of ancient impacts. Some believe that we should be looking in the sedimentary record and that many more impact craters remain to be found, hidden away in oil company seismic data.
In the last few decades geologists have recognised a range of distinctive features found in rocks that have suffered/enjoyed an impact. Structural features within the impact include shatter-cones, fractured rock and faulting. On a microscopic scale, features such as shocked quartz are distinctive traces of the sudden massive stresses of impact. For large impacts, the energy turns into heat that melts and vaporises rocks. If a big enough hole is made, there will be extensive metamorphic changes to deep rocks due to the pressure release of removing kilometres of rock.
Another way to identify past impacts is to find material thrown out beyond the crater area. This can include thick layers of fragments and glass, called suevite. Molten glass may be thrown up into space, falling again as spheres of glass over a wide area of the earth. These may be quite thin layers in sedimentary sequences. These surely represent the most extensive record of past impacts but not many have been recognised to date, so keep your eyes peeled. For recent impacts, the glass lumps may still lie on the surface, where they are known as tektites. The largest set of tektites is found in a vast ‘strewn field’ stretching from south-east Asia into Antarctica and yet no-one has yet the crater that was surely formed at the same time.
The hunt for impacts continues. In the last few months researchers have published evidence for two more. A huge old one in Greenland has long been eroded away, but there are lots of distinctive features in the deep rocks that sat below it, which is all that remains. A smaller more recent one in Canada shows contorted strata and lots of pretty shatter cones (fractures formed by the impact).

Shatter cones from Prince Albert crater. Courtesy of University of Saskatchewan. http://www.flickr.com/photos/usask/7644732242/in/set-72157630747745754/
The most inventive method of finding evidence of past impacts is to study ancient myths. Some link myths about ‘fire from the sky’ to impacts that occurred in human history and seek to explain flood myths by a cometary impact.
My awareness of my own geocentric bias makes me sceptical of such things. If I was an astrophysicist, aware of the sheer volume of space debris, I would be much more inclined to explain features of the earth in terms of impacts. Perhaps too much so. I read someone once trying to explain the South African Cape Fold belt as caused by the Vredefort impact. This was nonsense.
Of course this is how science progresses, by bringing perspectives from different disciplines together as a way of stimulating new research. The link between Chixulub and the K-T extinction is pretty clear (but still debated). What is interesting about many mass extinctions is that there are just so many plausible mechanisms. The Deccan Traps may have killed the dinosaurs just as much as a meteorite. In a similar way the end-Jurassic extinction is close in time both to an impact and extensive vulcanism. The only way to take this argument further is by careful study of the evidence, most of which sits in the rocks.
Study of earth’s early history removes any lingering doubts that earth can be studied in isolation from its surroundings. The earth formed within a dusty disc around the new sun 4.56 billion years ago. During the earth’s first few 100 million years it was constantly being struck by other pieces of debris. The best current theory for the formation of the moon is the ‘giant impact hypothesis’. This suggests that the proto-earth was struck by another proto-planet the size of Mars. The impact resulted in two separate blobs which formed the earth and the moon. The energy of such an impact left both bodies completely covered in a magma ocean. Any water in the earth would be boiled off, meaning that our atmosphere and oceans are all derived from water from comets that have hit the earth since. We are all made of star dust, but let’s not forget the comet juice.
My favourite link between the earth and beyond is only an idea so far, but a beautiful one. On earth we find rare meteorites that came from Mars and the moon. When one day we study the moon in more detail, perhaps we’ll find pieces of earth on there. The period when the most impacts hit earth (sending bits flying off) is also the time when we have the fewest rocks preserved on earth. What if the oldest earth rock still in existence is actually to be found on the moon?
To end, a look at earth from the outside to remind me that our planet, endlessly fascinating as it is, is only a tiny dot in space.
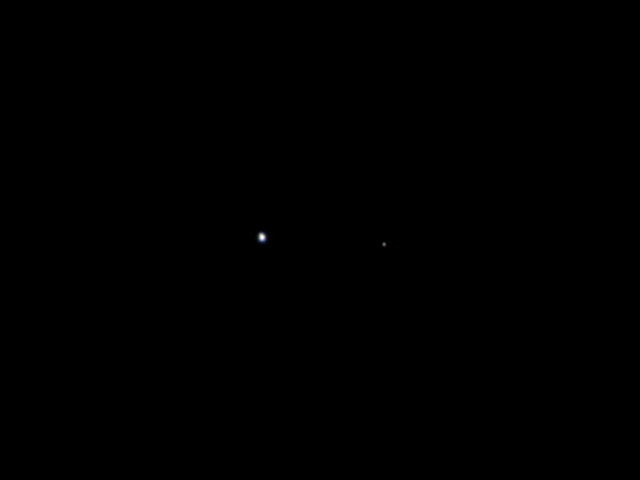
Picture of the earth and moon taken from Juno probe. Courtesy of Nasa http://www.nasa.gov/mission_pages/juno/news/juno20110830.html