A while ago, I asked Twitter for suggestions of topics for future posts. A great one came from Brian Romans, a Prof at Virginia Tech and a long-standing pillar of the online geoscience community:
How fast can rocks undergoing metamorphism be exhumed to the surface? How do we know (how can we measure/estimate)? https://t.co/qcsnxXKqhL
— Brian Romans 🌎🏔🌊🧊 (@clasticdetritus) December 24, 2016
This post follows on from my discussion of how quickly metamorphic rocks heat up which discussed an old conceptual model of metamorphism being caused by slow, gradual processes building mountains and heating the rocks inside. A more modern understanding shows that metamorphic events can be extremely fast, driven not by slow conduction, but rapid (even catastrophic) pulses of hot fluid or pressure.
Let’s think what happens next to the mountains in the old gradual conceptual model. They are slowly but surely brought low by erosion. Grain by grain of quartz or mica bidding farewell to their long-standing neighbours and heading off on an headlong tumbling adventure down a river to the sea. As the hills and mountains are laid low, once deeply buried metamorphic rock slowly emerges, cooling as it heads towards the surface over tens of millions of years.
Turns out, if you actually date the age of metamorphic rocks at the surface in modern mountain belts, they can be a lot younger than expected. Just as it doesn’t necessarily take millions of years to create a metamorphic rock, so they can reach the surface in double quick time.
How to date cooling
How do we know this? If you’re in an active mountain belt such as the Himalayas and you have a metamorphic sample in your hand, that’s already one piece of data – it’s at the surface and cool today. Date the age of the metamorphic event and estimate it’s temperature and you have a second data point. Those two together may be enough to demonstrate rapid cooling. But more data is always good. Luckily radiometric dating can really help us here.
Whenever geologists quote an absolute age1 for something, they are referring ultimately to an estimate derived from our understanding of radioactive decay. Certain elements (most famously Uranium) are unstable and their nucleus can fracture into pieces. Some of these pieces may be small and fast-moving – they make up the radioactivity that can be so dangerous – but there are larger fragments of nucleus too, which tend to stay put and are called ‘daughter elements’. They are the products of natural alchemy and form atoms of new different elements. The rate at which this radioactive decay occurs is steady and unchanging, perfect for a clock. Simply put2 if you measure how much there is of both the radioactive substance and its daughter, you can calculate the age of something. This is geochronology.
For us today, the key concept here is that ‘something’ we are dating the age of. Often we seek to find out the age at which a mineral grain grew, either in a metamorphic rock or crystallising from a magma. This works if the mineral acts as a closed system – once the grain is formed, nothing comes in or out. If instead some of the daughter element has left the system, then the date measured is ‘wrong’ – it doesn’t tell you the real age of the mineral. However, since the mineral becomes a closed system when it cools below a particular temperature3, careful measurements can give you the date when the mineral cooled below that temperature. This is therefore a new independent data point in the rock’s history of cooling. This is the principal behind the technique of thermochronology.
Different minerals, and different pairs of elements become closed at very different temperatures. Many common minerals are used to determine radiometric dates: biotite; muscovite and other white micas; various feldspars; garnet; calcite; apatite. The spread of closure temperatures is from around 1000°C (for Zircon) down to 70°C (for Calcite)4.
This gives us a very powerful tool kit. Different minerals in the same rock may give different ages, each dating a different stage in the rock’s cooling history.
Irish rocks are cool and once they were really hot.
Let’s move to an example of how thermochronology can give insight into how fast metamorphic rocks cool. It comes from a recent paper by Anke Friedrich and Kip Hodges focusing on Connemara in Ireland, (my old PhD field area). The paper cites my research, which immediately makes me think well of it.
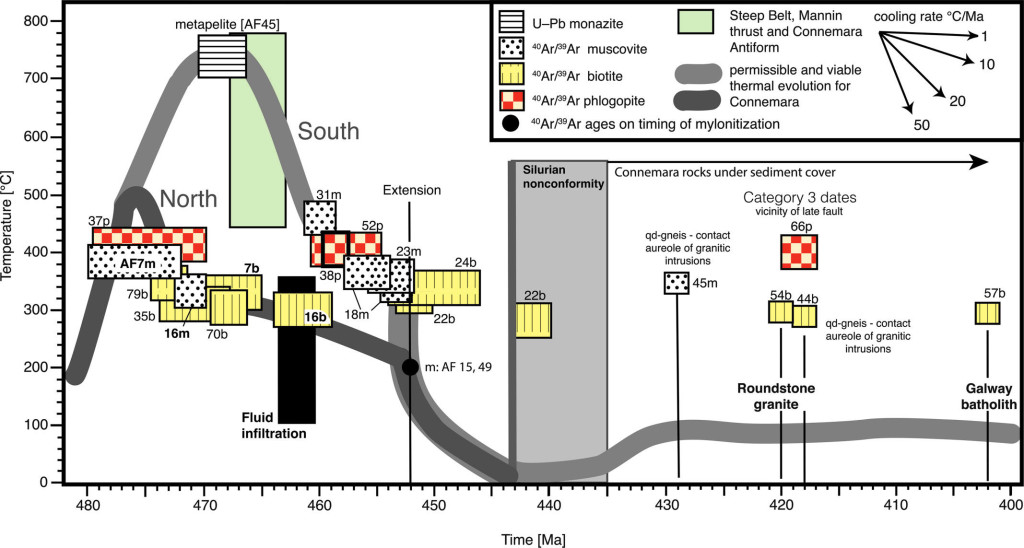
Figure 7 from Friedrich and Hodges (2016), showing the range of thermochronological dates and the contrasting T-t paths across Connemara.
This figure is the core of the paper. Each box is a different thermochronlogical measurement, plotted against time and Temperature. Different coloured boxes are different types of systems. Even the same colour of box can be at a different temperature because these estimates include also a consideration of the size of the mineral grain. The data is superficially rather noisy, and could be taken to indicate a slow cooling history, but the geology of the area is extremely well known, allowing distinct 3 sets of dates to be identified.
Those defining the dark line – labelled North – show a brief period of heating and then cooling. The south of Connemara was the site of igneous activity for much longer than the north – the later cooling is clear from the data. Data points from the right hand side of the graph are associated with heating from much later granites.
Scream if you want to go faster
Prof. Romans is a sedimentary guy and interested in metamorphic rocks as something that ends up as a layer of sand or mud. Linking metamorphic rocks to sedimentary ones means tracing out one of those great big linked cycles that make earth science so mind-expanding. My favourite example – good evidence for what went on deep in the Himalayas can be found in sediments deep in the Indian Ocean. As an aside, for these Connemara rocks we can also trace the point at which they start being eroded and ending up in a sedimentary basin. As a further aside, the grains that were dated from Irish metamorphic rocks can also be found in modern sediments. These studies (detrital mineral thermochronology) can be used to find out where sediment came from or to estimate the timescales of sedimentary processes. That’s a topic for another time.
Brian Roman’s original question started with asking how fast metamorphosing rocks can be exhumed. Exhumation means the rocks reaching the surface – like digging up corpses only much much nicer. This is linked to a rock’s cooling history as in order for a metamorphic rock to cool significantly and quickly it needs to get nearer the surface. Metamorphic rocks may form in unusually hot parts of the earth but the fact that the earth gets hotter the deeper you go is the main control. Places where rocks are exhumed quickly must be places where the rock above is removed quickly and one way to do this is to erode it away.
The fastest rates of exhumation known are in the Himalayas. Specifically at the eastern and western ends: Namche Barwa in the eastern Himalaya and near Nanga Parbat in the west. Here rocks are being drawn to the surface so fast that 25km of rock has been eroded in the last 10 million years. Given that the Himalaya are around 50 million years old and on average 5 kilometres high, this is quite amazing.
The likely reason for such rapid exhumation is that these points are where massive rivers, full of glacier melt from the Tibetan plateau and Himalayas, cross the Himalayan mountain range. The rivers cut deep down into the earth and soft hot rocks flow into the space created (more detail here). The Himalayas and the Tibetan Plateau cause the Monsoon which drives rain-clouds north from the ocean to feed Himalayan glaciers. Melting glaciers fill the rivers, which cut deep into the earth and drive a flow of rock from kilometres under the ground, up to the surface where it is turned into sand and mud that flows back down into the ocean. There it forms sedimentary rocks, that one day may be buried and be turned back into a metamorphic rock. There are so many cycles in geology and so many links between them.
In the first post in this series we learned that the formation of those beautiful metamorphic minerals paraded on Twitter every #thinsectionThursday is less like the slow transformation of dough baked into bread but instead more like the sudden explosive transfiguration of a hard kernel into tasty pop-corn5. In this post we’ve learned how we know how quickly rocks cool. This can be slow, like letting a roast chicken rest before carving but other times it’s like popping some morsel straight from the fryer and onto your plate