Fifty kilometres is not far. World-class marathon runners run 42km in a little over 2 hours. They only move along the earth’s surface though. Getting to 50 kilometres below your feet is a different thing entirely. It’s a place of crushing pressure and meltingly high temperatures, somewhere human beings will never go. There is a type of rock that’s been there – and deeper – and yet somehow returned to tell the tale. On this journey its been transformed multiple times, released fluids that cause new crust to be generated far above, even grown diamonds. Its also the most attractive rock type of all. It’s called eclogite and its gorgeous.
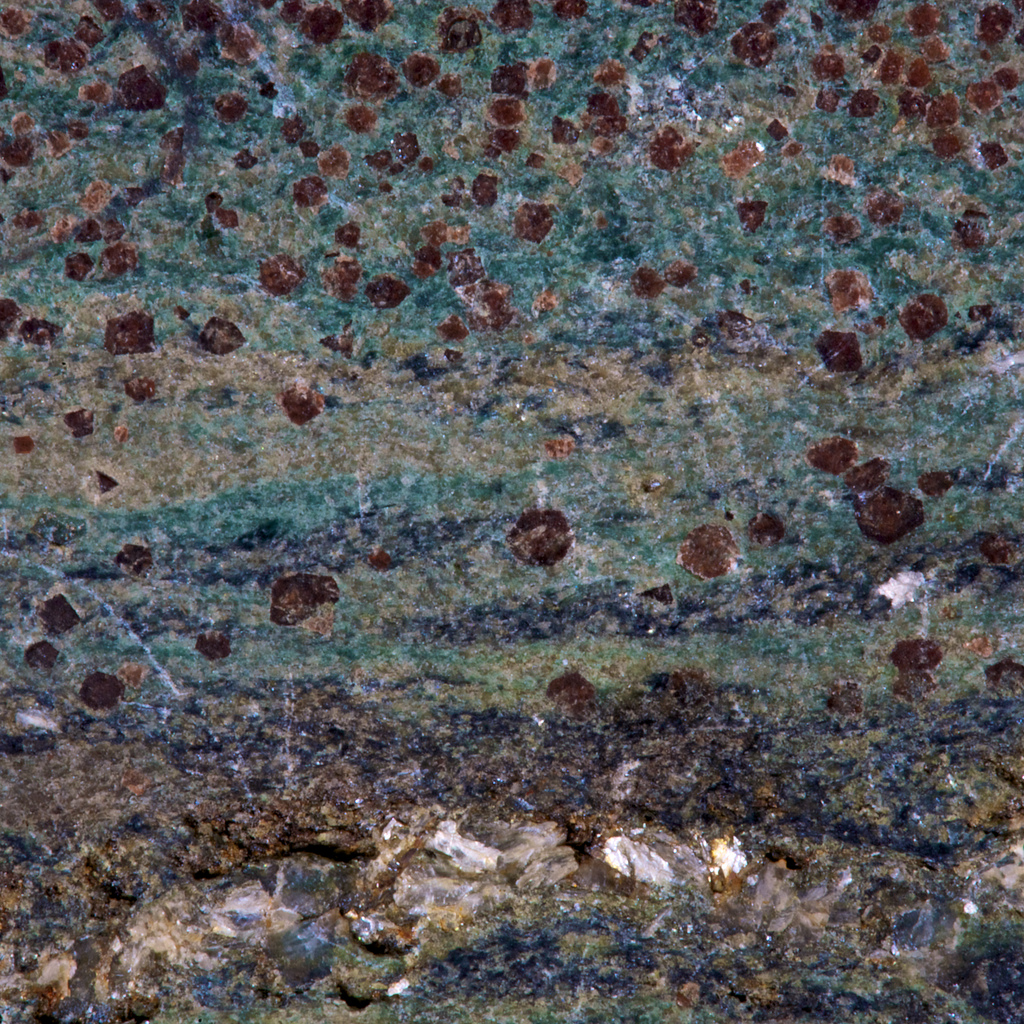
This is from the lawsonite type locality, Reed Station on the Tiburon Peninsula, Marin County, California. Keele collection. From @hypocentre on Flickr http://www.flickr.com/photos/hypocentre/4639062593/in/photostream/
The beautiful red mineral is garnet, the lovely green is omphacite, the exquisite blue glaucophane.
Eclogite is rock of mafic composition (igneous rock relatively low in silica) that has been buried to 50 kilometres or more. At this depth, everyday minerals such as plagioclase feldspar or augite are no longer stable and they break down, allowing the rock to grow new denser (more attractive) minerals that are better suited to higher pressures.
Eclogites are typically formed by subduction of oceanic crust. Oceanic crust forms at mid-ocean ridges, where melting of the earth’s mantle produces a layered crust of mafic composition, basalts on top, gabbro below. New areas of oceanic crust are constantly being created and, to balance, older colder oceanic crust is pushed back down into the mantle at subduction zones. As the crust is pushed deeper and deeper, the gabbro and basalt is transformed into eclogite.
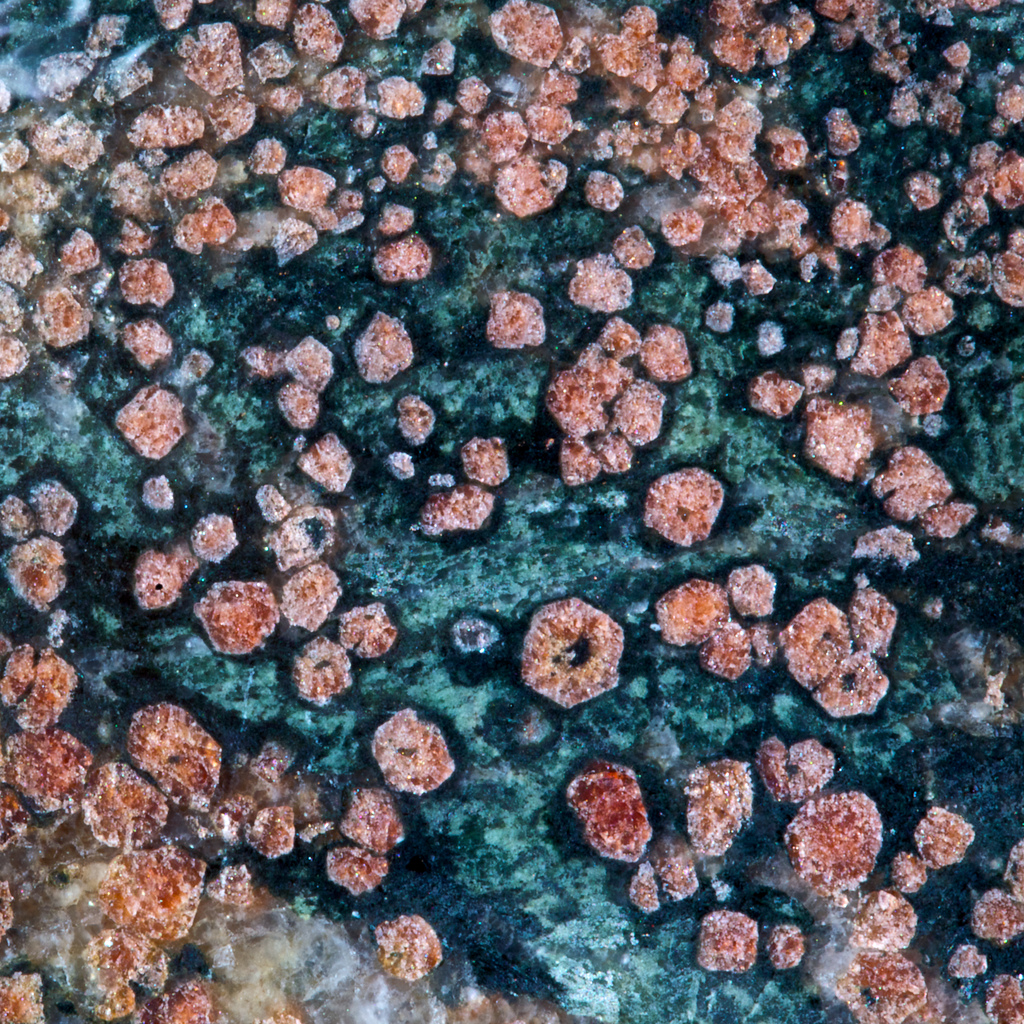
Another picture from Ian Stimpson. Sample from the Mariánské Lázně Complex in the west Czech Republic. Keele Collection. http://www.flickr.com/photos/17907935@N00/4652324838
Pieces of eclogite that we can study and admire have somehow been brought back to the surface. How does this happen? Does subduction go into reverse, or does it get back to the surface in some other way? I’ll save these questions for another post.
Most eclogite doesn’t reach the surface, but returns to the mantle for good. Eclogite is denser than the surrounding mantle rocks and some subducted plates reach the very bottom of the mantle nearly 3000 kilometres below our feet. Material that’s been on this journey started as humble basalt but has been through a whole range of transformations, into eclogite and beyond.
For this post, I want to stick with a paper that illustrates how eclogites provide direct evidence of processes that we could only otherwise study indirectly.
Deep earthquakes, those below about 70km depth, are a little mysterious. At those depths, rocks are so hot they flow rather than fracture and so squeezed that fractures shouldn’t be able to move. Deep earthquakes are only seen in subducting slabs of oceanic crust. There’s been much speculation about the possible mechanisms that allow deep earthquakes. This is mostly based on seismic evidence and our knowledge about the transformations that occur in these rocks as they are buried. Wouldn’t it be great to somehow get hold of actual rocks from one of these deep fault zones?
Tucked away in the Italian Alps the Monviso Ophiolite is a crustal slice of an extinct ocean called Tethys. As if moving from ocean basin to high in the Alps wasn’t enough, it’s also been buried 80 kilometres under the surface. As described by Samuel Angiboust and colleagues in this months Geology, it contains not just eclogites, but eclogite breccias, something never found before. These are not sedimentary breccias – the material between the angular blocks is not sand or mud but consists of eclogite facies minerals. The eclogite was fractured at great depth. Even individual grains of garnet show evidence of multiple episodes of fracturing. There is a lot of deformation going on, there is a mylonitic fabric, a result of ductile deformation (flow rather than fractures), active both before and after the fracturing.
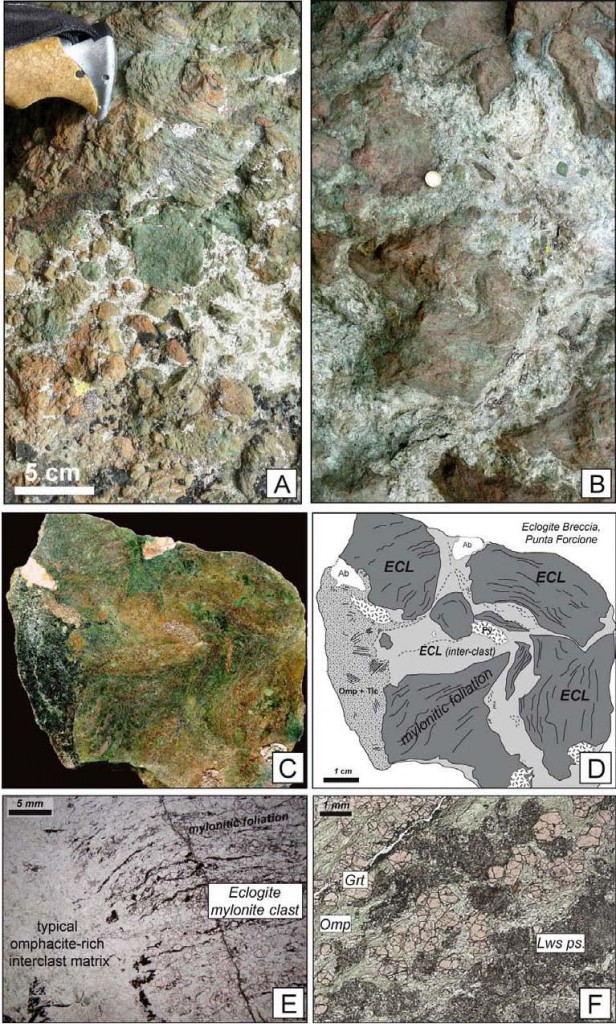
Typical textures from eclogite breccias described by Angiboust et al. Figure DR-1 from DATA REPOSITORY/ G32925 (URL in text). Follow this link for more description.
Using multiple lines of evidence, our authors suggest that the breccia was formed in a fault zone that created Magnitude 4 earthquakes at between 70 and 80 km depth. The minerals in the breccia suggest fluids were present and may in some way have allowed the fracturing to occur. The other forms of deformation (mylonites and fractured minerals) were relatively dry, but also silent and not directly detectable from the surface.
Eclogites are like mysterious and beautiful emissaries from another world. You can learn a lot about a place remotely, by listening to its earthquakes and so on, but finding something that’s been there and can tell you about it – that’s really special.
References
S. Angiboust, P. Agard, P. Yamato, & H. Raimbourg (2012). Eclogite breccias in a subducted ophiolite: A record of intermediate-depth earthquakes? Geology DOI: 10.1130/G32925.1
Supplementary material found at DATA REPOSITORY/ G32925 (downloadable PDF).