Cratons are pieces of continents that have been stable for a over a billion years. As earth’s plates drift along, mountains periodically rise and fall, plate boundaries appear and disappear. But cratons are like great-grandmothers at family gatherings, while younger crust moves excitedly around them, they sit quietly, occasionally remarking on how different things were when they were young.
Every continent has cratonic areas, notably the core of North America, Scandinavia, Siberia, India and most of Australia. They may be covered by a thin shawl of sediment, but often they expose ‘basement’ rocks such as gneiss. Economically they are very important – most of the world’s diamonds come from cratonic areas as do many other valuable deposits.
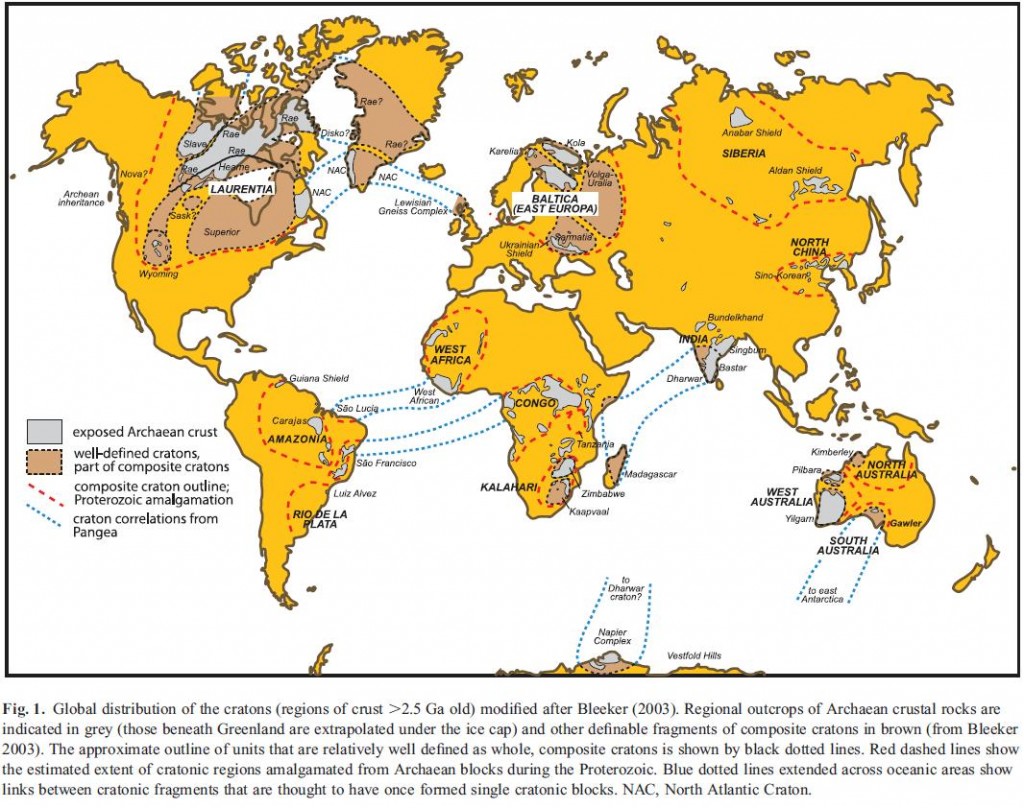
Cratons are stable because they are strong. The geology of the Himalayas illustrates this – the modern day plate boundary between Indian and Asia is at the southern edge of the Himalayas. The cratonic Indian plate is barely deformed, in great contrast to the vast pile of deformed soft young crust in the Tibetan Plateau to the north.
Cratonic crust is strong, being unusually cold and dry, but that is only part of the picture. Continental crust is the upper portion of continental lithosphere. It’s lithosphere that puts the plates into plate tectonics, it’s a rigid layer on the earth’s surface, as opposed to the hot flowing mantle that lies beneath (the asthenosphere). Lithosphere consists of the crust and an underlying layer of mantle that has become ‘stuck on’. This layer of lithospheric mantle can be 2 to 3 times thicker than the crust above and so contributes a great deal to the strength and stability of continental lithosphere.
It turns out that the lithospheric mantle beneath cratons is unusual. In oceanic crust, as it ages it cools and eventually it sinks down into the mantle again. It is thought that continental lithospheric mantle can fall off as well, but in cratons this doesn’t happen. Finding out why is an interesting challenge but before we start that journey, a brief interlude….
Geochemical interlude
My feelings about geochemistry have changed over time. As a doctoral student I was exiled out into an annex 10 minutes from the main department because a new piece of geochemical apparatus required a lab to be expanded, swallowing our little rock-filled office. I could relate to metamorphic petrology and analysis of mineral chemistry, but the isotope stuff, seemingly requiring months of juggling Hydrofluoric acid to get a couple of data points, left me cold. Years later, with a broader, wiser perspective I find myself marvelling at the way isotope geochemistry leads to so much awesome science.
There are two flavours of isotope geochemistry, stable and radiogenic. Many chemical isotopes are unstable and over time spontaneously turn into different elements, the bits left over being thrown out as matter and energy – radiation. For geologists, this real-life alchemy, known as radioactive decay, is mostly used to tell how old something is. The rate of radioactive decay is constant, so measuring the current abundance of isotopes and working backwards tells you the time elapsed since an event happened. Getting from measurements to age involves assumptions about the sample which may be incorrect – my own research successfully predicted a published age was incorrect. Nevertheless modern geochemists have a wide range of extremely robust and accurate techniques at their disposal. Datable events include a crystal forming from a magma or growing in a metamorphic rock, a surface being exposed to the atmosphere, a critter growing, a mineral cooling below a particular temperature, when magma was extracted from melting rock, even the age of ground water.
Stable isotope geochemistry relies on the fact that two isotopes of the same element, for example Hydrogen and Deuterium, are not precisely the same. Most physical and chemical processes treat them identically, but some do not, the slight different in mass means slight differences between isotopes. For example during evaporation of water the lighter H216O will vaporise first, creating a difference in isotopic composition between the water vapour and the remaining liquid. This phenomena of isotopic fractionation affects water in other ways, such that there is a relationship between fossil oxygen isotopes (in ice or fossil sea shells) and the temperature of the sea they came from. This tremendously useful technique is just one example of the seemingly magical way tiny differences in the physics of atoms can shed light on diverse geological problems. There are many other applications, but the technique shines most when we have very little other evidence to go on. Research into the formation of the earth and moon relies heavily on studies of arcane isotopes. We have only limited information about lithospheric mantle from beneath cratons (remember them?) and isotope geochemistry tells us many interesting things…
Back to the cratons
A recent review by Cin-Ty A. Lee, Peter Luffi, and Emily J. Chin of Rice University focuses on the creation and destruction of continental mantle (I’ll mostly discuss creation).
If lithospheric mantle beneath cratons was the same composition as the rest of the mantle then over time it would cool and become denser and unstable. A consensus has emerged that it does not because at some point in the past it was involved in melting and up to half of its volume was removed. The rock left behind after the melt flowed away is known as depleted peridotite and it is stronger and more buoyant than normal mantle, so it remains stable for billions of years.
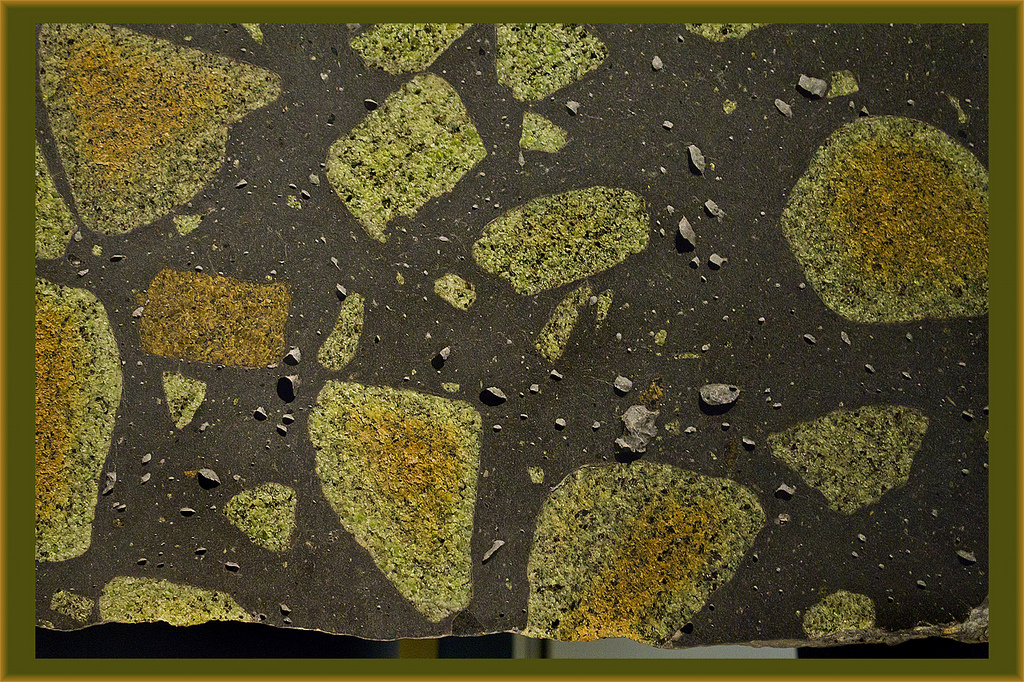
Fragments of mantle peridotite in lava.
Pieces of continental lithosphere do reach the surface, sometimes as huge slabs such as at Ronda in Spain. Some weird volcanic deposits start at mantle depths and bring up pieces of the surrounding rocks. Sometimes they contain diamonds, but also pieces of the lithospheric mantle. Armed with these samples, geochemists have applied a massive array of techniques.
The geochemistry of melting rock is well understood and it allows us to infer many things about the conditions under which it happens. For example comparing ancient with modern samples, we can tell that older samples melted at higher temperatures (1,500 to 1,700◦C 1,300 to 1,500◦C) and saw more melt extraction (50 to 30% versus <30%). This is consistent with the fact that the early earth was hotter.
Melting is affected by pressure as well as temperature – deeper mantle rocks contain different minerals (at these pressures, garnet at depth, spinel above) which melt in subtly different ways. Cratonic peridotites melted at shallow depths (90km) but were later moved deeper (180km).
Not all samples are of peridotite, next common are pyroxenites, sometimes referred to as eclogites. Chemically these are similar to basalts formed at modern mid-ocean ridges. Their oxygen isotopes show wide variation, such as is seen in oceanic crust following hydrothermal alteration. Other physico-chemical processes could in theory cause similar patterns, but it seems only low-temperature processes explain the patterns seen. The fact that they were once part of oceanic crust makes these pyroxenite rocks very different from the peridotites (which have only ever been part of the mantle).
Further evidence that pyroxenites were once near the surface comes from sulphur isotopes from eclogitic inclusions within diamonds. Fractionation of these isotopes occurs in ways only explained by chemical reactions high in the atmosphere. These diamonds with eclogitic inclusions themselves have carbon isotopes that are extremely ‘light’. Light carbon is a sign of life – organisms preferentially contain light carbon. In these ancient rocks, life probably means bacteria. So we can lengthen the list of “lovely things bacteria help to make” – cheese, soy sauce, wine, yogurt, healthy digestion and now certain types of diamonds.
NB for visitors from the German wikipedia page on Diamonds, I’m delighted you’re here. Here is a better reference for the science on this.
This is a wonderful thing. A bacterial mat sitting on the sea-floor billions of years ago ends up being stuffed down into a subduction zone. It enters a world of crushing pressure and extreme temperature where it is transformed utterly, its carbon forming a diamond. Later a weird eruption happens and the diamond is squirted back up to the surface for us to marvel at. Only isotope geochemistry (and some generous assumptions on my part) allows us to tell this story.
How does continental mantle form?
Our authors discuss how continental mantle forms (spoiler: we don’t really know). One model is that a large plume of hot mantle material rises up underneath a continent. This would indeed form lots of depleted peridotite (underneath a big pile of lava). However this model is inconsistent with the evidence described above – melting would be expected at greater depths (200km) than is seen.
A plume model also fails to explain the eclogitic parts of the mantle. One mechanism to explain how portions of oceanic lithosphere end up in the sub-continental mantle is that when subducted instead dropping steeply down they remain buoyant and stack up beneath the continents. In this model, the sub-continental lithosphere grows by progressive capturing and stacking of oceanic lithosphere. Its been argued that parts of the Farallon plate were captured beneath western north America in recent times. Hotter oceanic lithosphere (common in the Archean) subducts at a shallower angle and so is more likely to end up getting stuck to the base on the contintent.
This model explains the evidence for shallow melting and subsequent burial, plus the presence of former oceanic lithosphere. Indeed it predicts a larger proportion of eclogitic pyroxenite than is observed. Our authors propose a process of “viscous drainage” whereby the crustal portion of the stacked ex-oceanic lithosphere (now dense inclined sheets of garnet pyroxenite) slowly ‘drains’ down and out, leaving the peridotite ‘framework’ behind.
Models of the creation of continental crust emphasise the importance of island arcs. An oceanic island arc (where oceanic crust, subducts under oceanic) may collide with a small continent, turn into a continental island arc and ultimately become a new piece of continental crust. This mechanism will not give thick lithosphere on its own however – so some process of ‘orogenic thickening’ (squeezing it so it’s thicker) is required.
You will perhaps have sensed that the paper drifts into speculation a little. This is appropriate in a review paper and inevitable as we are on the ragged edge of what we can know about these rocks, so distant in space and time. The paper ends with a ‘future directions’ section full of as yet unanswered questions.
“How do continents form?” is a simple question to ask but we still can’t give a complete answer to it. We still don’t know the secret to great-grandma’s longevity.
Peridotite picture from dun_deagh on Flickr under Creative Commons.
Craton map from Pearson and Wittig under Geological Society of London fair use policy.
PEARSON, D., & WITTIG, N. (2008). Formation of Archaean continental lithosphere and its diamonds: the root of the problem Journal of the Geological Society, 165 (5), 895-914 DOI: 10.1144/0016-76492008-003
Lee, C., Luffi, P., & Chin, E. (2011). Building and Destroying Continental Mantle Annual Review of Earth and Planetary Sciences, 39 (1), 59-90 DOI: 10.1146/annurev-earth-040610-133505