Since ancient times in China, special glass stones have been recognised. Called Lei-gong-mo, or “Ink sticks hurled from the sky by the thunder god” these are now known as tektites. These remarkable stones are found on the surface in a small number of areas (called strewn fields) around the world.
There are various types of tektite such as splash-form, ablated and layered. They are all lumps of extremely dry glass, often with distinctive shapes.
There is a trade in tektites and you can buy them on eBay. I’m tempted, but I gather fraud is a problem and I can never get over a feeling that they look like cat-poo.
How are they formed?
Tektites have been recognised for thousands of years. Geologist Charles Darwin* thought they were volcanic in origin, not unreasonable since volcanoes produce lots of glass. By the mid-Twentieth Century they were thought by many to be blebs of glass that had fallen through the earth’s atmosphere. Ablated tektites in particular suggest this. Look at the picture below- the central top area is the top of an originally spherical blob. The base was then melted again by friction as it fell through the earth’s atmosphere, the material being pushed up and around to form the distinctive shape. One idea was that the material originally came from the moon before falling to earth, but this was ruled out once actual lunar samples were collected: the geochemistry just didn’t match.
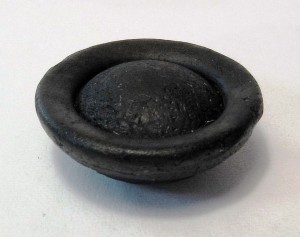
Aerodynamically shaped / ablated tektite (http://en.wikipedia.org/wiki/File:Australite_back_obl.jpg)
Tektites are now seen as resulting from large impacts on the earth’s surface that throw molten rock into space where it falls back, covering large areas. They are found in distinctive areas, strewn fields, nearly all of which have been associated with an impact crater. Within a strewn field, the age of the glass and the impact match, plus there is a size distribution, with large chunks near the impact and smaller tektites further away. Micro-tektites, also known as spherules (a purely descriptive name) may be found enormous distances away. Layers of micro-tektites are now being found in sedimentary rocks, particularly in the Archean (when impacts were much more common).
A recent paper by Kieren Howard in the Proceedings of the Geologists’ Association (doi:10.1016/j.pgeola.2010.11.006) provides a nice overview of modern thinking on tektites, drawing on numerical modelling of impacts. Melting of rocks within an impact crater is no surprise, the energies, and therefore temperatures and pressures produced by the impact are extremely high. What is less obvious is that melting happens in different ways. As the shock wave from the impact passes through the ground it can produce large volumes of melt. Most of this is mixed up with the fractured country rock to form melt breccias (pseudotachylites) and some is mixed up with rock fragments and thrown out to form a layer near to the crater (suevites). The rocks closest to the impact reach temperatures >5000°C, producing a mixed melt+vapour phase. This is ejected in high velocity jets travelling at ‘cosmic velocities’ (>11 km/s) that push away our planet’s puny atmosphere in a process called atmospheric blow-out. This means that small volumes of melt can effectively fly into space and then fall to earth – tektites!
Tektites are very very dry (< 0.05 wt % water) which is attributed to a process called “bubble-stripping”, where the water is lost as vapour under decompression. The dryness therefore is not related to the conditions under which the melt formed. Indeed Kieren Howard’s main argument is that tektites are especially common in impacts where the surface layers are particularly moist. He believes that the presence of water near the surface means that large volumes of especially high velocity melt can be produced.
Case of the missing crater
The biggest strewn field on the planet is the Australasian. This stretches from SE Asia through to Antarctica and is robustly dated at 0.803 Ma. The only problem is nobody knows where the crater is! An impact spread 10 million tons of glass across a huge area, but the only trace found to date is the tektites themselves. The usual pattern of size distribution in the strewn field points to SE Asia – there are lots of large (>1kg) tektites in Thailand, Cambodia and Vietnam but the expected big circular hole in the ground is not to be found. It is likely therefore that the impact was offshore and the crater has since been buried by more recent sediments. Another possibility is that the impact was oblique, at a shallow angle, and that there is a structure to be found that isn’t a classic circular crater. As you can imagine, the hunt is on, and Kieren Howard is part of it. Once he, or someone else, finds the source of these tektites, expect to hear about it. This is surely a story to capture the imagination of non-specialists (like journalists).
Drifting slightly off-topic, a related paper in Geology in 2008 made me the most jealous I’ve been for a long time. It documents finding micro-tektites in Antarctica, extending the Australasian strewn field even further (>400km). The authors sampled isolated mountains, surrounded by the Antarctic ice-sheet (nunataks). Basically they picked-up whatever sand was lying on the surface or tucked into joints and found little beads of glass – micro-tektites – in it. These landed 800,000 years ago and yet are just lying there waiting to found. Tektites in other areas, Australia say, have been buried and then uncovered, pitted by weathering and such-like. But in Antarctica nothing has happened for nearly a million years (or longer). Erosion is low because of very low precipitation and there are no other sources of sediment to bury the micro-tektites. They fell out of the sky and then nothing happened, until some Geologists came by. Other studies, using cosmogenic nuclide dating have shown that some rock surfaces in Antarctica are 2 or 3 million years old. This astonishes me every time I think about it, places like this just don’t feel like they belong on earth. Just to make that clear, the surface is 3 million years old, not the rock itself but the surface you can put your hand against. What’s more, scientists going to such places are the first human beings ever to go there (unless other scientists have been there before). I’m used to places that were scraped clean by glaciers 12,000 years ago, and continuously inhabited ever since. Visiting these other-worldly places on the very bottom on the world must be a very special experience indeed.
References
“Tektites in the Geological Record, Joe McCall, Geological Society of London, 2001” is a little old now but contains lots of details on tektites around the world, including all the detailed evidence supporting the impact origin of tektites.
http://tektites.co.uk contains lots of information and lovely pictures and is the best place to go next if you want more tektite fun.
Notes
* I know, I know, he is rather better known for his role in biology, but his training was as much geological as biological. Also the concept of Deep Time was vital to his development of his theory of natural selection. Without a knowledge of the geological evidence that the earth is rather older than 6,000 years, Darwin’s insight that species evolve due to small incremental changes doesn’t really make sense. Plus he was the first to explain the formation of coral atolls.