This post is part of my journey into the geology of mountains.
Rocks can behave rigidly, or under some conditions, they are ductile and can flow. This is a remarkable thing, which helps explain why the theory of Continental Drift was not more widely accepted (prior to the plate tectonic revolution of the 1950s). Seismic evidence from the deep earth shows that the crust and mantle rocks behave elastically (on short timescales). This was taken as evidence that they could never flow, meaning the continents couldn’t drift. We now know that hot rocks in the mantle can flow (over long timescales) and that this is a necessary part of plate tectonics.
What about other geological implications? What other things can be explained by the ‘Jekyll and Hyde’ way in which rocks deal with stress?
Small scale structures: Most rocks under surface conditions behave rigidly – they only change their shape by fracturing or by being dissolved. Rocks deformed near the surface are therefore typically fractured or faulted. Slightly hotter rocks may be deformed by dissolution, where material is dissolved into solution and redistributed into veins. Stylolites and cleavage are structures formed in this way that are common in deformed sediments. Salt and mud and are two substances that can flow in cold shallow rocks. Salt diapirs and mud volcanoes are structures where these light substances flow towards the surface.
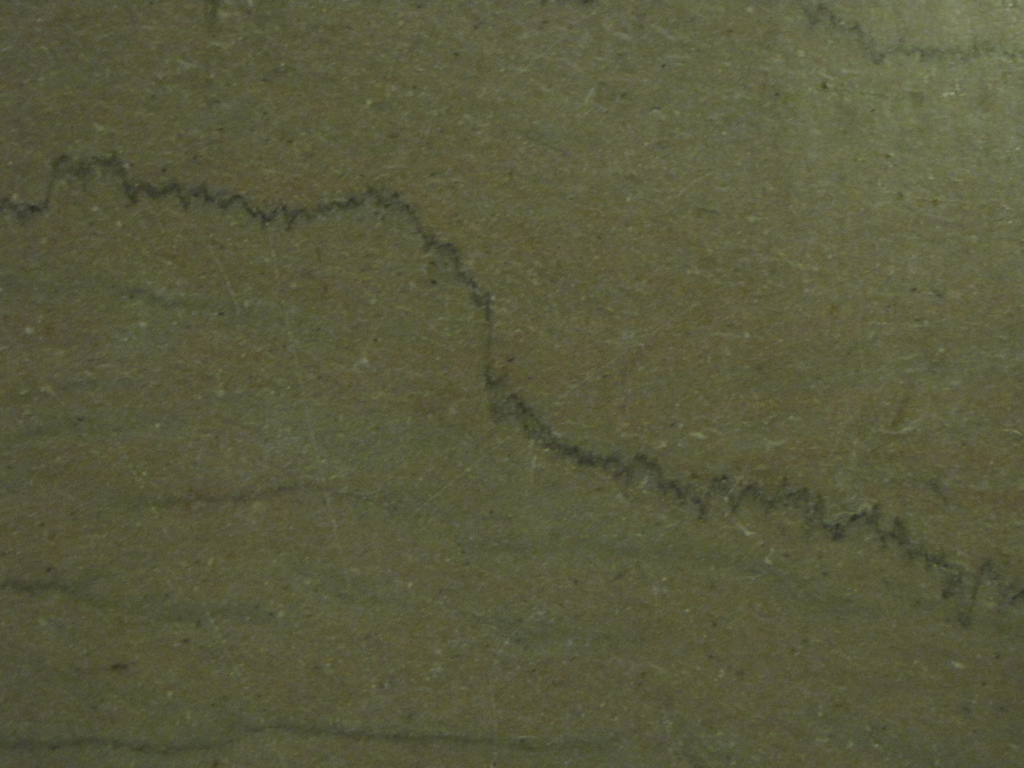
Stylolite from Ron Schott on Flickr (http://www.flickr.com/photos/22644739@N00/4468019786)
To deform typical rocks in a ductile way, they need to be under conditions associated with metamorphism – below depths of about 15-25 km and above temperatures of ca. 300 oC.
Under these conditions, various forms of sold-state creep begin to work and rocks can ‘flow’. Typical structures here are fabrics, such as schistosity or gneissosity – entire rocks are deformed as shown by the flattened minerals within them. Often there are areas of very high strain called shear zones, typically containing mylonites. A process called strain softening, plus perhaps a focussing of water or heat flow makes these zones softer than the surrounding rocks, meaning that they get more and more deformed.
Shear zones are analogous to faults, indeed a major structure may be a fault near the surface but a shear-zone at depth. A shear-zone is likely to be wider than the equivalent fault – the deformation is distributed through a large volume of rock.
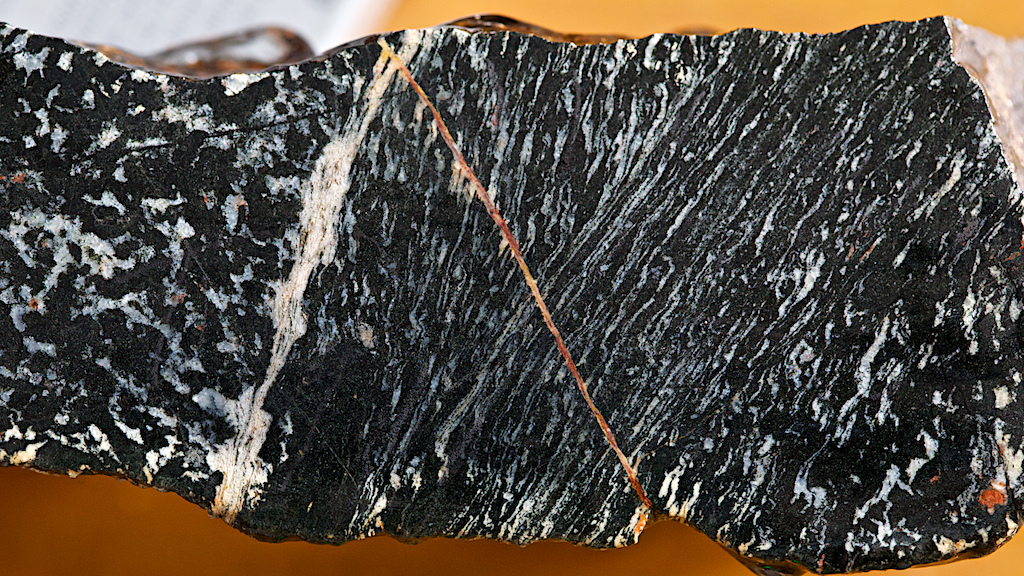
Shear zone in amphibolite from Ian Stimpson @hypocentre (http://www.flickr.com/photos/17907935@N00/6723121739)
What controls the brittle-ductile transition? Major controls on the rheology of rocks are composition, temperature, pressure, the presence of liquid, and the presence of melt. Composition is quite hard to change, but if you increase any of the other things, the rock is more likely to behave in a ductile fashion.
Large scale features, large scale evidence. There are some big features that can be explained in terms of their rheology, which means they can be used to directly measure the rheological properties of the crust.
Foreland basins are sedimentary basins where the accommodation space (the hole in the ground filling up with sediment) is created by the flexure of the rigid upper crust. Think of the Indian continent being pushed under the Himalayas and Tibet. The weight of Tibet is pushing down on the Indian plate and this bends it downwards. The space created fills with sediment being eroded from the Himalayas. The Ganges basin is formed.
The Indian crust is cold and rigid. Clever folk can do the maths on the shape of the crust as it bends down. This confirms that the pattern matches the model for rigid, elastic deformation. It also allows then to calculate the plate’s flexural rigidity, which is a measure of its strength. This means quantifying the rheology of real bits of the earth, which is a very useful trick.
If you read up more on foreland basins, the picture gets more complicated as, over time the crust will further deform in a viscous way. I want to take you away to a different example of viscous behaviour.
There are raised beaches all over high-latitude areas of the Northern hemisphere. These are fossil beach deposits now found metres above sea-level. They can’t be explained by global sea-level changes as equivalent features are not found further south.

Raised beach from Scotland (in foreground). From SAGT @http://www.flickr.com/photos/sagt/4304338029/
These raised beaches formed when global sea-level was similar to today’s level: they are raised because the earth’s surface has risen up since they were formed. This isn’t due to tectonics – these areas are far from any plate boundary. What these areas have in common is that they were covered with kilometres of ice during the last ice age. This ice weighed a lot and this force pushed the crust down. The upper crust bended rigidly, pushing underlying viscous rocks (probably in the mantle) out of the way. The ice melted relatively quickly, removing the downward force. The viscous mantle rocks are slowly recovering, flowing back into glaciated areas and lifting the earth’s surface, leaving fossil beaches high and dry. The overlying rocks are being passively pushed up, with occasional post-glacial earthquakes occurring in otherwise tectonically quiescent areas. Once again, these natural phenomena are an excellent opportunity to directly measure the physical properties of the earth’s interior: the rate of rise lets you measure the viscosity.
Crème brûlée versus jelly sandwich
So the earth is strong near the top and weaker at deeper levels? If so, it would be like a crème brûlée. A giant spoon digging into the earth would crack through an upper layer (like burnt sugar in a crème brûlée) and then scoop gently through the soft mantle beneath (creamy custard).
If the earth were boringly simple, this would be accurate, but as you know, life is more interesting than that. The earth gets hotter with depth, so rock is more likely to be weaker with depth, but the composition of the earth is not constant. It’s long been known that the surface layer that moves as part of plate tectonics (the lithosphere) is not just the crust. There is a layer of mantle material (lithospheric mantle) stuck to the bottom of the crust meaning that within the lithosphere, there is a dramatic compositional change which complicates matters.
An interesting paper by E.B. Burov and Tony Watts (available here, thanks GSA!) discusses the crème brûlée model and compares it with a ‘jelly sandwich’ model. A jelly (jam) sandwich has a soft layer between two stronger layers. The sandwich model supposes a strong rigid upper crust, a weak hot lower crust and then a strong layer of lithospheric mantle.
You’d have thought that, knowing the composition of average crust and mantle and the conditions with depth, we could resolve this debate easily. As it turns out, although we know that olivine-rich mantle is much stronger than quartz-rich crust we don’t know by how much. It is very hard to run experiments with low strain rates and high temperatures comparable to real-life conditions so we can’t measure directly.
Burov and Watts combine numerical modelling and indirect observations to settle the issue. Think back to the Indian crust subducting under Tibet. One of the things you can infer from geophysical studies of these situations is the effective elastic thickness of the lithosphere. This is the cumulative thickness of rocks that are behaving elastically. For India, this is 70km, which is greater than the thickness of the crust. This is hard to explain, unless the lithospheric mantle is strong, suggesting you have a thick sandwich, not a thin layer of burnt sugar. They also show numerical modelling that suggests that without a strong lithospheric mantle, subduction and mountain building wouldn’t be possible.
Note that for this last section I’ve switched to talking about weak/strong rather than rigid/ductile. If you a simple sort like me, you can think of the two as synonymous, but they are not really which is why Watts and Burov talk about long-term strength. Complications arise because most rocks are viscoelastic – they transmit earthquake waves elastically (over short timescales) but behave viscously over longer timescales. The paper gives you a taste of this, but I’m not the man to take you any further into that world.
Rocks behave in complicated ways when placed under stress. I hope I’ve given a taste of the complications that arise from this in the wider world. I leave you with one thought: if you squeeze an over-filled sandwich – what happens to the filling? I will return to this and how it relates to Tibet and the Himalayas, in another post.