Structural geologists seek to understand how rocks have changed shape, in order to better understand wider processes such as how mountains are formed. Sometimes they use a terminology called ‘Deformation-numbers’ which I will now explain via a series of pretty pictures.
Structural geologists spend their day measuring the orientations of things. These can be planar things, like sedimentary bedding, fault planes, cleavage planes and other metamorphic fabrics; or linear things like fold axes or mineral stretching lineations. All these things interact in various ways, but given time and a compass-clinometer a good geologist will work it all out.
The trouble is that rocks are complicated. Take these gorgeous pics from northern Norway, kindly provided by Stephen Daly.
It’s clear that there is tight folding in metamorphosed sediments. There are things to measure the orientation of, such as hinge line (where the fold is tightest) and the axial plane, (the surface joining the hinge lines, here flat lying).
But, as so often in field geology, a closer look from the same area reveals a more complicated picture. What can you see here?
There is a clear set of folding – the obvious wavy pattern of the dark and light layers. Try tracing the individual layers – they are not even. In fact there are two sets of folding visible in these rocks. Let’s trace it out.
The orange lines are the axial traces of the obvious set of folding. The straight blue lines are axial traces of another set of folding. The curved blue lines are the faint traces of folded sedimentary layering.
This rock has enjoyed two phases of folding – therefore any description of the deformation in such complicated rocks has to introduce the concept of a sequence of events. Geologists love this sort of thing. I remember a seminar in the 1990s about the first detailed images of topography from Venus, showing linear structures. The lecturer said that as a geophysicist his first reaction was to perform a mathematical analysis of their spacing, but that a geologist’s first reaction was to look for cross-cutting relationships. This fundamental geological instinct applies to folds as well. One of the ‘blue’ folds is clearly folded by an ‘orange’ fold, meaning that ‘blue’ is older than ‘orange’.
A simple way of expressing this is to label the folds F1 and F2. The smaller the number, the older the structure. The same applies to other types of structures – a planar structure is know as S, starting with bedding which is know as S0. In our example S0 is folded by F1 and F2. A metamorphic fabric formed at the same time as F1, but folded by F2 would be S1. Most likely F1 and S1 were formed by the same deformation event, which we would call D1.
An Irish example
Another thread through much of geology is scale. Let’s move away from the outcrop scale to one of kilometres. Here is a classic cross-section through the Connemara, in Western Ireland.
If you give brilliant structural geologists enough time and enough Guinness, this is what you get. This is from the classic 1979 paper on Connemara by Tanner and Shackleton (1979). The different shades of grey each present a different group within the Dalradian Supergroup, each of which contain distinctive layers of sediment. This varied package of sediments allows the complex folding to be worked out.
In blue I’ve highlighted the trace of the Derryclare anticline, a tight structure that folds the sediments associated with a phase of deformation know as D2. In orange I’ve highlighted a few later D3 folds that contort the Derryclare anticline. Note that these are themselves bent over by a major D4 structure that covers the whole of Connemara.
On an outcrop scale in Connemara, most outcrops show clear D3 folding as in this rather splendid marble outcrop.
In Connemara, a view on the kilometre scale is the best way to see D2 and D4 structures, but sometimes a closer look is best. If you glue a slice of rock to a piece of glass, grind it down to a very thin slice and shine light through it then you can look at it under the microscope. This is another way of finding structures, more often fabrics than folds.
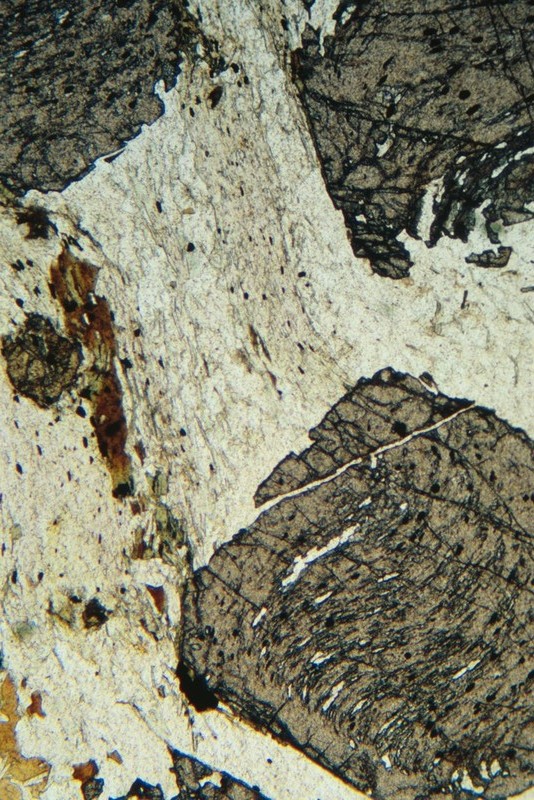
Photomicrograph of garnet-mica schist. Image courtesy of British Geological Survey Geoscenic archive
The image above is of a deformed schist. The large grey lump is a garnet crystal, a porphyroblast that grew during metamorphism. Look at it like a structural geologist – what do you see? I see this:
The orange lines show a fabric within the main body of the rock, visible in aligned quartz and mica. This is likely to correspond to the fabric visible in a hand specimen of the rock. In blue I’ve sketched a fabric visible only in the garnet. As the garnet grew it swallowed up fragments of quartz and other minerals that were themselves flattened into a fabric. This older fabric is now preserved only in the rigid garnet. Outside the older blue fabric has been destroyed, partly by metamorphic recrystallisation, partly by a later deformation phase that squashed the minerals to form the orange fabric
Wider implications
Looking at thin sections allows us to find fine structures that may no longer be visible in an outcrop or a hand specimen. It also let’s us link metamorphic and structural histories together. In our thin-section example the garnet (or at least its core) was growing while the older structure still existed, that is before the later orange deformation episode. If we found a mineral that grew across the orange fabric, it is probably younger than it.
I hope you can see that this allows us to build up two mutually-supporting sequences of events, the structural and the metamorphic. Metamorphic events are sometimes referred to as M1 and M2 and correlated with deformation events. Once you’ve done this, its possible to start linking D and M numbers to tectonic events: an arc colliding with a continent, for example.
Careful now!
Discovering one fold twisting another is an observation. Talking about D1 and D2 and then correlating that with rocks kilometres away is an interpretation. Geologists are excellent at carefully turning multiple observations into rigorous interpretations, but there are various reasons to treat them with care.
The first one is that modern models of mountain building processes (where many deformed rocks form) emphasise gradual processes. The continuous readjustment of an orogenic wedge, maybe switching into channel flow and out again, all this predicts a bewilderingly complex sequence of events for the whole orogen. The second related issue is that a single outcrop may not preserve the entire structural history. Structural and metamorphic processes will not affect an entire mountain belt at once – deformation and metamorphism may be focused into particular areas (perhaps rich in heat and water) and leave the surrounding rocks untouched. Maybe correlations of deformation episodes over wide areas are simply wrong. Maybe ‘D2’ is consistently a strong deformation followed by ‘D3’ folding, but these events happened at different times in different places?
There’s an analogy here with the study of separate sedimentary basins. In the absence of dateable fossils, the age of a sedimentary basin may be poorly known. Even so, geologists will attempt to correlate separate basins based on events preserved in the rocks – we’ve got to do something, even if we know the correlations may be incorrect. If a dateable fossil is found, it may show us we’ve made a mistake, but more likely the sedimentary history and correlations will make the fossil more useful. It doesn’t just tell us the age of a particular layer, but by inference it can illuminate the history of a much wider suite of rocks.
Within deformed metamorphic rocks, we can’t use fossils, but we can use the isotopes within minerals to tell us the age of events. For a while we’ve been able to do this accurately for zircons, but recently we can also directly date metamorphic minerals. Sometimes, for example in Connemara, the metamorphic dates are consistent with a single sequence of structural and metamorphic events that can be linked to arc-continent collision. However increasingly detailed studies of many areas are finding that apparently similar fabrics were formed in different mountain-building episodes, millions of years apart. Single grains of garnet have been found that contain a core that grew hundreds of millions of years earlier than the rim1
The concept of deformation sequences, as a set of observations, is invaluable for linking a particular isotopic age to a wider tectonic context. To say that a particular mineral grain grew at a particular time is not in itself very interesting. But it seems that interpreted correlations of D numbers without isotopic dating should be treated with care.
I’ll be illustrating these concepts with a specific example in my next post on the great Dalradian D2-D3 controversy and my part in it.
References
Argles, T., Prince, C., Foster, G., & Vance, D. (1999). New garnets for old? Cautionary tales from young mountain belts Earth and Planetary Science Letters, 172 (3-4), 301-309 DOI: 10.1016/S0012-821X(99)00209-5
Tanner, P., & Shackleton, R. (1979). Structure and stratigraphy of the Dalradian rocks of the Bennabeola area, Connemara, Eire Geological Society, London, Special Publications, 8 (1), 243-256 DOI: 10.1144/GSL.SP.1979.008.01.25