Driving west across the edge of the English Peak District is a good way to see how geology shapes landscape. Tracing the routes that cross it – feeling their shapes with a finger on a map or with your body as the car swings round bends – hints at how they are shaped by the landscape beneath, but also the intentions of the people who first made them. Paths across the Cheshire peak were shaped by dramatic changes across both human and geological history.

The new Buxton road winds below Shining Tor. © Copyright Jonathan Wakefield and licensed for reuse under this Creative Commons Licence
Roads in Derbyshire’s ‘White Peak’ are shaped by the limestone beneath; they sit in the bottom of ‘dales’ – steep gorges etched into rock – or wind across a bucolic landscape of green fields tessellated by white stone walls. But drive out of Buxton on the Macclesfield (“Cat and Fiddle”1) road and you suddenly enter a wilder world. Within a few feet, the stone walls at the side of the road turn from pale grey to a buff beige. The landscape is brown and open, empty under a sky that is rarely entirely blue, mantled by peat bog and growing little but heather. You are entering one of the ‘Dark Peak’, one of the wild moors of northern England, the wuthering heights where Heathcliff roamed and Ted Hughes’ hawk roosts.
Further west, at the edge of the moors everything changes again – the Cheshire Plain appears laid out for inspection. On a clear day – or better still night – the view takes the breath away. The homes and lights of 3 million people twinkle and beguile. The depth of detail invites you to study, to pick out Jodrell Bank, flights descending into Manchester airport, Alderley Edge….
Drivers shouldn’t enjoy the spectacle: this road needs your full attention. It’s popular with motorcyclists for its many bends. Sadly some are total idiots, making the A537 one of Britain’s most dangerous roads. Their attitude to the area is not much different from many other modern travellers – this is a place to enjoy yourself in. Older generations – those who made this and other routes – had other motivations.
Early trade-routes
Some old routes over the high moors of northern England are know as the Saltways. The ‘wiches’ of Cheshire: Nantwich, Northwich and Middlewich, are towns based on salt. Thick layers sit deep within the Cheshire Basin, formed as a shallow sea was repeatedly evaporated under the Permian desert sun.
Salt has been produced in Cheshire since at least Roman times. An important commodity essential to food preservation (cheese! bacon!) it was transported across the country by salt traders (“salters”) who name attached to their routes. Below is my inference as to the route taken by salters through this area, passing through Saltersford Hall.
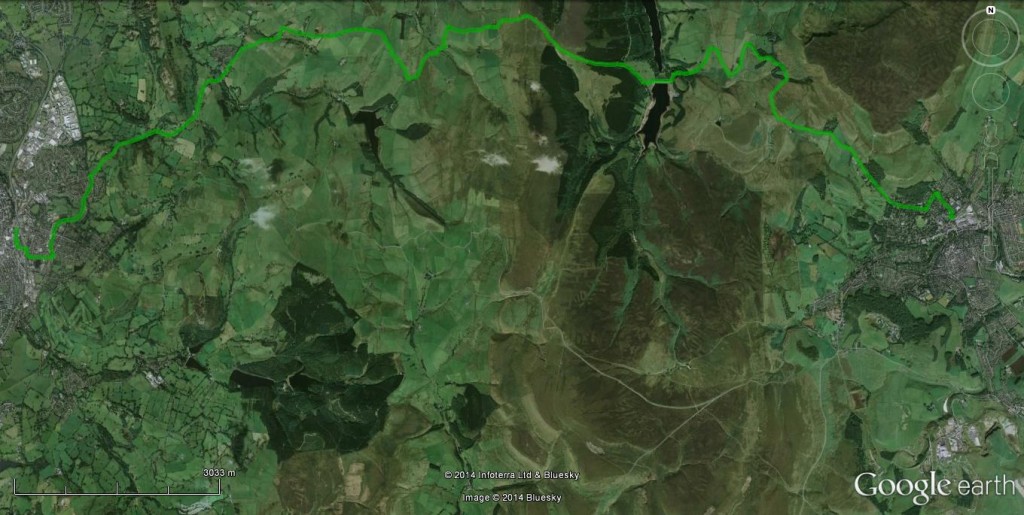
Trace of Salters way in green. Macclesfield is the town on the left, Buxton on the right. The brown area in the middle is the moor
Buxton was a Roman town and a direct line from the salt towns to there passes this way, but there is not good evidence it is that old. These routes are pre-industrial though, used by men and horses walking through the landscape, at the mercy of the elements. A reminder of how perilous this could be – hard to remember when speeding in a warm car – comes from an odd memorial stone on the route, that reads: “Here John Turner was cast away in a heavy snow storm in the night in or about the year 1755. The print of a womans shoe was found by his side in the snow where he lay dead”

The front of the memorial stone. Source
The modern age approaches
Even as John Turner grew cold in the snow, the epoch-making2 Industrial Revolution was hotting up. Using new technology to centralise production in factories only make sense if you can then get your goods to the people who buy them – new forms of transport were an important factor.
The 18th Century – early on in the Industrial Revolution – innovation came in the form of many new roads, called turnpikes. These were independently financed toll roads, sanctioned by Acts of Parliament.
The first Macclesfield-Buxton toll road followed an old route, was ‘engineered by a blind man, John Metcalfe’, and opened in 1759. Initially controversial, it was opposed by some (local coal producers) but championed by the new industrialists.
This road and the salters way are both direct but steep. This is ideal when moving goods with pack horses, but horse-drawn wagons work better with more gradual slopes, even if the route is longer. By the dawn of the 19th Century, new road-building techniques had emerged that cut into the hillside to make wider carriage-ways that avoided steep slopes even over hilly terrain.
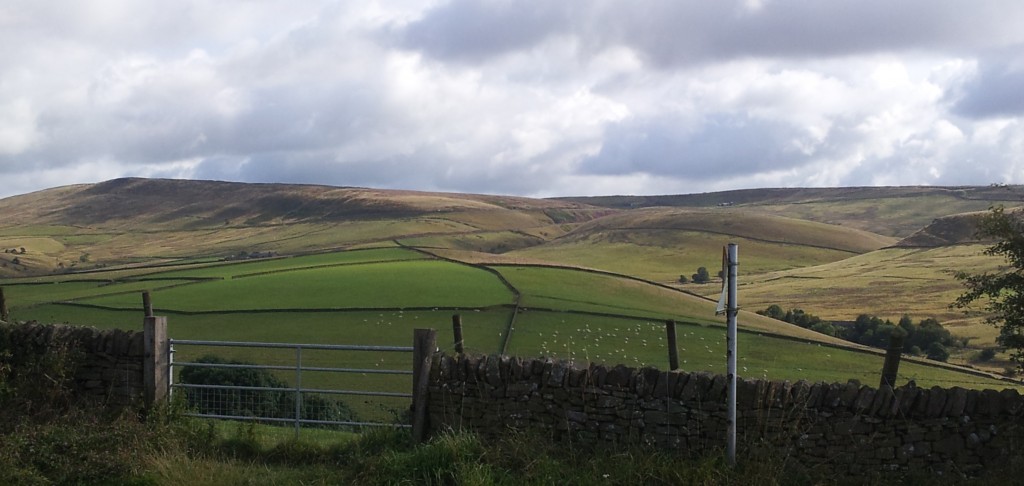
New and old Buxton roads converge on the far hillside. The Cat and Fiddle pub is on the right hand skyline
In 1808, a new Eddisbury bypass just above Macclesfield was built by the famous engineer Thomas Telford, the “Colossus of Roads”. In 1821 the rest of the Macclesfield-Buxton road was modified with new winding flatter routes and a pub for the weary. The new road is wider than the old and climbs more gradually. To achieve this is has many bends, which attract the loonies in leather on their motorcycles.
A new ancient road
The transition from White to Dark peak, as you go east from Buxton is dramatic to us, but the incoming darkness would have been felt much more keenly by the Carboniferous inhabitants. The sparkling tropical seas where trilobites frolicked in crinoid forests were suddenly snuffed out by the arrival of massive amounts of sand and mud. Rocks made from the remains of life are replaced by those where fossils have to be sought out – traces in sand, crushed shells in rare marine muds or eventually, coal.
The most modern path across the Peak is also the most ancient. Walking across these hills for pleasure is extremely popular and paths easily cut into the soft peat. The most popular routes are now paved with big slabs of the local sandstone – along Shining Tor there are hundreds of them. Covered in ripples and the traces of burrowing bivalves, walking along these makes you feel like you are on a sandy shore 300 million years ago.