Look at a large-scale geological map and, provided the area is not covered in recent sediments, there will be large areas of red showing outcrops of granite. There are many ways in which rocks can be melted to produce granitic magma. But it’s long been recognised that there is a ‘space problem‘: how do those big red areas on a map form? How does the molten rock get there and where has all the rock that used to be there gone?
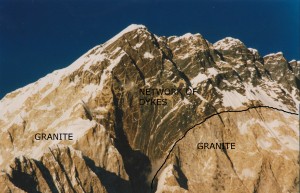
How did all that granite fit into there?
The easy stuff
Let’s start with the easy explanations. First, intruding magma near the surface. There are a range of structures – sills, dykes, laccoliths, and ring dykes – that are associated with intrusion of magma at shallow depths. All of these features involve magma moving along a plane of weakness (a fault or bedding in sedimentary rocks) and forming a relatively thin layer. Making the space is no problem – the magma can simply push the surface up. Satellite imaging of volcanoes often shows the surface rising up and down as magma moves about so no problem here.
Large areas of granite are more common at greater depth. If there is 10km of rock above, it is not obvious how the magma could push the surface up. Also a typical granite intrusion forms an oval shape on the surface – how does such a shape form?
Inadequate solutions
Papers on plate tectonics sometimes sketch out things in a cartoony way. For island arcs, where they want to show the extensive granite intrusions, people often draw as a blob, with a little tail at the bottom. It’s only a cartoon, but it conveys a popular but incorrect idea – that granite magma ascends as a diapir.
In sedimentary basins it’s not uncommon for thick layers of salt to become unstable and for some to rise towards the surface as a blob-like diapir, like wax in lava lamp. For a long time it seemed plausible that light granite magma moved up through the crust in the same way. It would certainly explain the round shape of intrusions. However granite can only flow when it is hot. Unless it is in the lower crust (or perhaps in hotter Archaean crust), doing the maths shows that any granite diapir would cool and freeze long before it got anywhere.
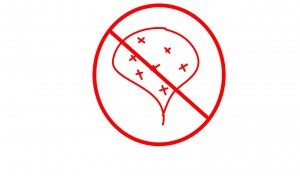
Just say no to granite diapirs
Another concept that doesn’t quite explain how granite intrusions form is stoping. This is the process where blocks of the surrounding ‘country rock’ fall into the granite and are sometimes melted. Granites are often rich in such fragments (called xenoliths), but it is only a marginal effect. The vast majority of a granite intrusion is granite brought in from elsewhere – stoping doesn’t help explain how space is made for it.
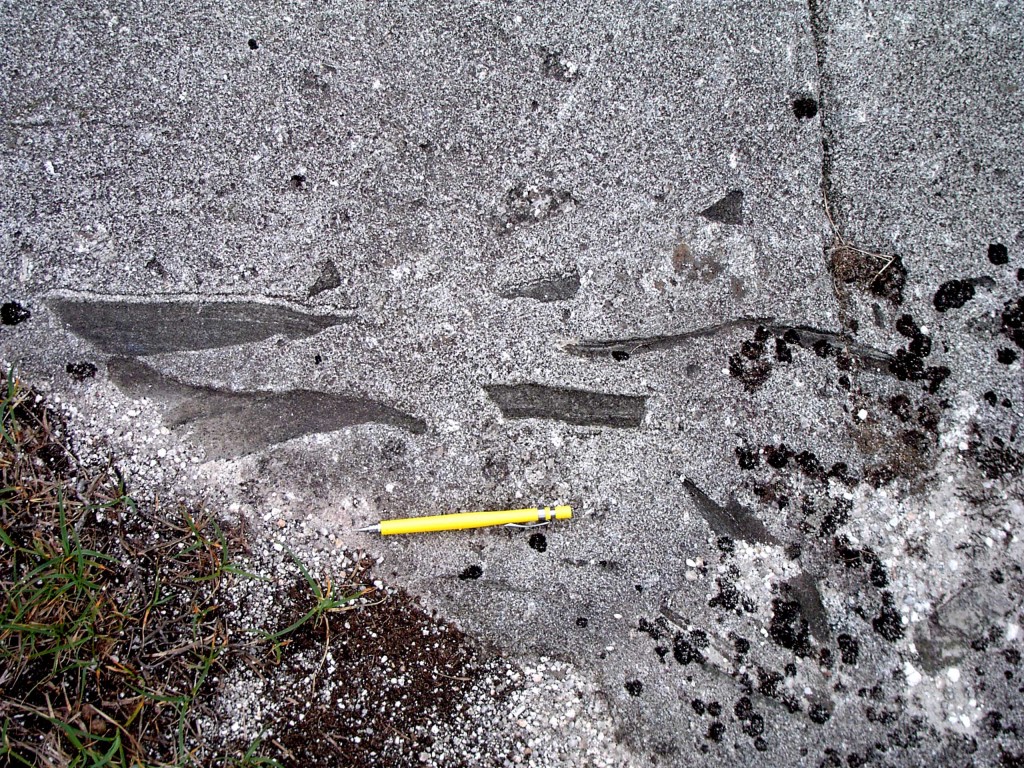
Granite from Donegal. Xenoliths in microgranite near Polcrovehy. Courtesy of Carl Stevenson.
Modern ideas
Melting of rocks to form granite magma is more common in the lower crust (it’s hotter down there). This is a continuous process and it turns out that it is fairly easy to squeeze out the magma, even if it only forms a small percentage of the melting rock. Rock-melt mixtures are very soft and easily deformed. When this happens, the magma is easily squeezed out and being light and under pressure, it moves its way upwards.
Part of the reason for the popularity of the diapir model was the thought that granite magma was too sticky to flow up dykes the way basaltic magma does. Basaltic magma on the surface (where we call it lava) flows beautifully, whereas lava of granitic composition flows only reluctantly – instead it tends to get stuck in the volcano which eventually explodes.
More modern studies of the viscosity of granite magma show that within the middle crust, granite magma can easily flow up vertical cracks. It can do this extremely quickly and it can happen with small volumes of magma.
It turns out therefore, that all these great big red areas on geological maps are actually made up of many small batches of magma joined together, either as separate sheets or by mixing within a magma chamber. This makes sense – it more closely matches the record of surface vulcanism – volcanoes don’t grow overnight but through a series of many eruptions.
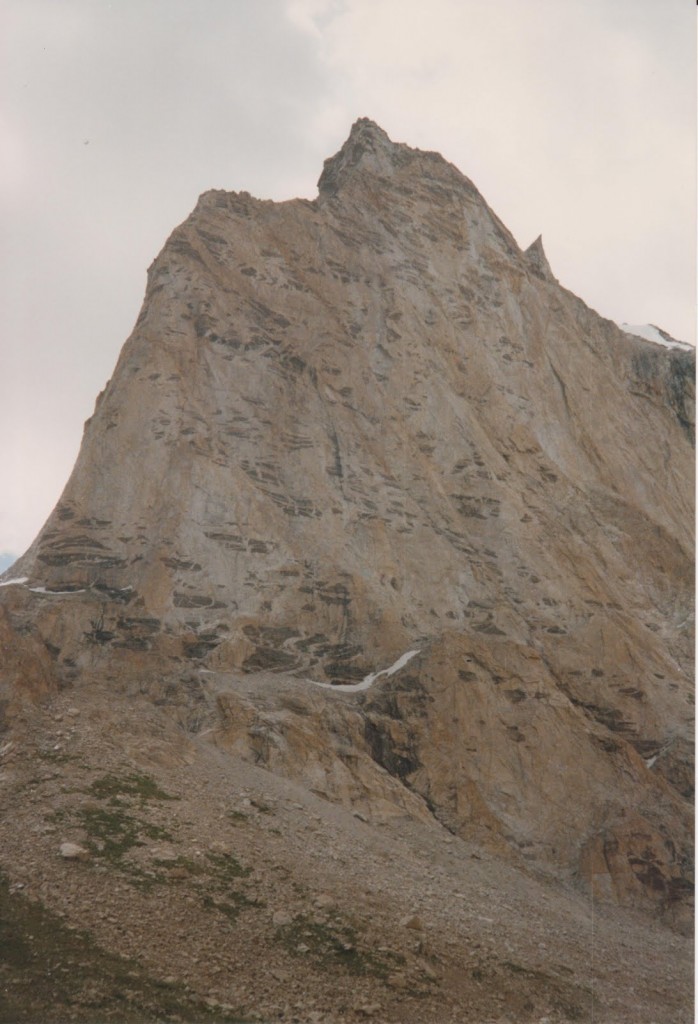
Complex granite intrusion, with dark xenoliths. Gumbaranjon, Ladakh, India.
How do large intrusions form through multiple batches of magma? Just like shallow intrusions, they tend to make use of existing structures. Only in the middle crust these aren’t brittle structures and sometimes they are still moving as the granite arrives.
A good way of illustrating all this is to go to Ireland.
Irish men of granite
Before we get to the rocks – an interlude. For me the best granites are Irish for personal reasons. Much of the best research into granite emplacement has involved graduates of Queens University, Belfast. Donny Hutton, John Reavy, Ken McCaffrey and Carl Stevenson were all trained there and have worked on Irish granites. John Reavy taught me and I’ve encountered all the others in some way or other: for me granite is best pronounced with an Ulster accent.
To drift off on a tangent a little, a story John Reavy told me about being a trainee geologist in Northern Ireland during ‘the Troubles’. Belfast city centre was regularly bombed by terrorists during this time. Fancy buildings would be seriously damaged, their polished stone cladding (including granite) broken into pieces and flung about the shattered streets. Enterprising geologists would then pick up some nice samples, once the dust had settled. Life goes on, after all.
To Donegal
Back to the rocks.

Map of Donegal’s granites. Taken from Stevenson et al. (2007)
The granites of Donegal are classics. In the 1980s, a series of papers by Donny Hutton laid out the link between the granites and deformation of the surrounding rocks. A key finding was to show that the granite magma was intruded at the same time the surrounding rocks were being deformed. This was a neat trick, partly because at the time there was no way of dating deformation directly. Dating intrusions however, was possible.
Sometimes the Donegal granites were deformed after they became solid rock. We can tell this because all mineral grains are affected and some have flattened shapes.
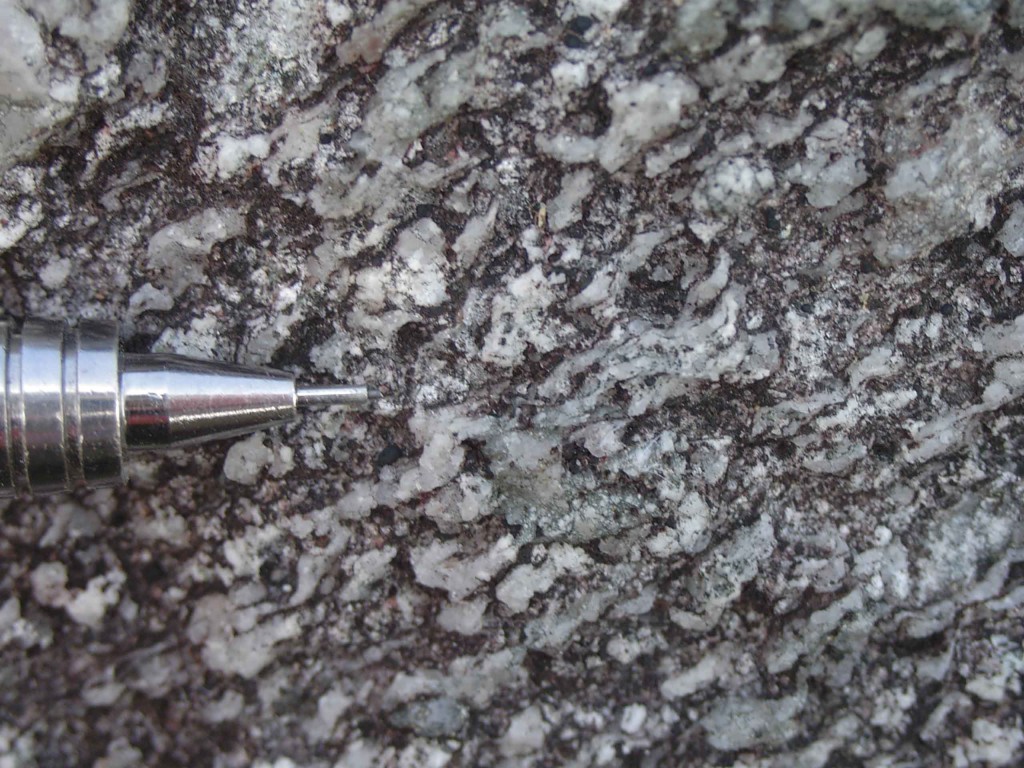
Strong ‘solid state’ fabric in Donegal granite, note the flattened quartz grains indiciating deformation of solid granite. Photo courtesy of Carl Stevenson
Some of the granite was deformed while partly liquid. In this case all the mineral grains look perfectly normal, only some structures are aligned. Minerals that form early in the crystallisation process may be aligned but are themselves undeformed – they were rotated as a crystal-melt mush was squashed.
Using such techniques, Donny Hutton showed that the Donegal granite formed within an active shear-zone. This led to a period of 10 years where pretty much every granite pluton in the British Isles had its intrusion linked to active structures. If rocks are deforming there are lots of ways in which space can be made for granite intrusions to form.
State of the art
When I was doing my undergraduate mapping in Donegal, the easiest bits to map were the granites. Granite was granite, as far as I was concerned – easily identified and quickly marked on the map. This was fine for that level, but with enough work, it’s possible to distinguish between individual batches of magma by clocking subtle differences in mineralogy or grain size. There’s a beautiful example of this from El Capitan in Yosemite.
Another technique tells you more about the structures within the granite. Some granites are rich in structures – aligned minerals or composition variation, you can find examples in many places. However granites that look featureless may contain minerals that are aligned. A technique called “anisotropy of magnetic susceptibility” or AMS allows us to measure the orientation of magnetic grains within the granite. This can pick up fabrics even in weakly deformed granite (or other materials).
Applying these techniques to the Donegal granites allows a detailed picture to be built up.
The Main Donegal Granite (MDG) marks the site of a major crustal shear zone that provided a route of ascent for multiple batches of granite magma. It now is filled by sheets of granite, often deformed, that entered space created by dilation of the shear zone. Some batches of granite that ascended this way ‘leaked out’ of the shear zone.
One of the leaked batches ‘ballooned’ out, pushing the surrounding rocks out to form the circular Ardara intrusion. Other leaking batches intruded less forcefully, forming horizontal sheets (called laccoliths) that thicken out in domes to various degrees.
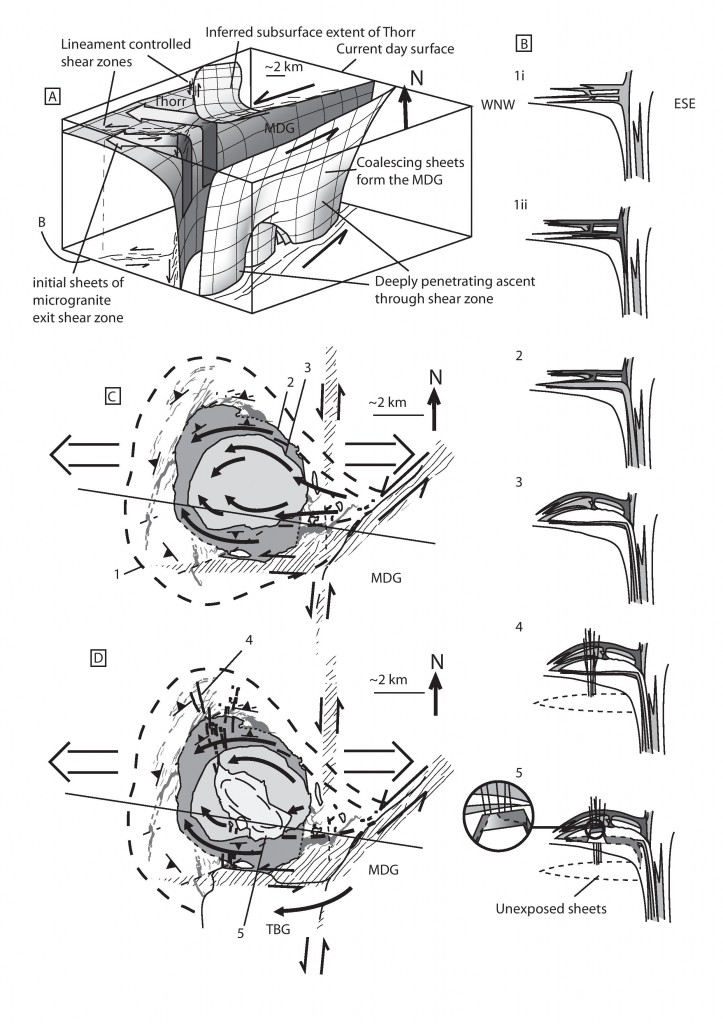
Diagram showing interaction between ascent and emplacement of Donegal granites. From Carl Stevenson
The space problem solved
The Donegal granites demonstrate two processes that are of global significance: how granites ascend and how they are emplaced. Vertical structures allow granite magma to flow up in small batches. At a particular level in the crust they migrate sideways and are emplaced, often as horizontal sheets.
By using existing structures and building up intrusions via small batches, there is no need to massively deform the surrounding rocks, the way diapirs would have to. Looking at a map view of granites, a sea of red, it is hard to think where the rock that used to be there has gone. As often in geology, the answer is to think in 3 dimensions – the rock was either pushed up (and has since been eroded) or was pushed down under the granite. Thinking about the crust as a whole, moving granite magma from the lower crust into higher rocks doesn’t involve a significant change of volume. Material melted out of the depths creates a sheet in the middle crust, pushing down the intervening rocks.
A theme of granite intrusion studies from Ireland and Scotland is the importance of pre-existing structures, particularly active ones. In detail, two sets of structures are important – the main MDG shear zone and a nearly-N-S lineament, that is believed to be linked to a major crustal structure.
Work on the equivalent rocks in Scotland (by Jacques and Reavy) presents a regional model where the lower crust is made up of a series of ‘lozenges’ formed by the intersection of two sets of structures (one set related to contemporary plate tectonic movements, the other pre-existing). Granite intrusions are predominantly found where these structures intersect. Movements along them may have influenced the siting of melting, the ascent of the granite and also their emplacement.
The problem of how granite intrusions form has long been known of. The tale of how it was solved is a typically geological one, requiring the synthesis of many techniques and the application of ‘four-dimensional thinking’. A problem best seen in a geological map can be solved by thinking how rocks move vertically and change over time.
References
Stevenson C.T.E., Hutton D.H.W. & Price A.R. (2006). The Trawenagh Bay Granite and a new model for the emplacement of the Donegal Batholith, Transactions of the Royal Society of Edinburgh: Earth Sciences, 97 (04) 455-477. DOI:
Petford N., Cruden A.R., McCaffrey K.J. & Vigneresse J.L. Granite magma formation, transport and emplacement in the Earth’s crust., Nature, PMID: 11130061
Stevenson C. (2009). The relationship between forceful and passive emplacement: The interplay between tectonic strain and magma supply in the Rosses Granitic Complex, NW Ireland, Journal of Structural Geology, 31 (3) 270-287. DOI: 10.1016/j.jsg.2008.11.009
JACQUES J.M. & REAVY R.J. (1994). Caledonian plutonism and major lineaments in the SW Scottish Highlands, Journal of the Geological Society, 151 (6) 955-969. DOI: 10.1144/gsjgs.151.6.0955